Research - (2022) Volume 10, Issue 8
Antibacterial Activity and Cytotoxic Effect of Bisphosphonate Conjugated Gold Nanoparticle Synthesized using Asparagus racemosus Root Extract
Sruthi H1*, Navaneethan R1 and Rajeshkumar S2
*Correspondence: Sruthi H, Department of Orthodontics and Dentofacial Orthopaedics, Saveetha Dental College and Hospital Saveetha Institute of Medical and Technical sciences (SIMATS), Saveetha University, India, Email:
Abstract
The nanoparticles can be surface modified to attach a variety of ligands which enable them to be biosensors, molecular-scale fluorescent tags, imaging agents, and targeted drug delivery vehicles. Green synthesis of nanoparticles is a type of bottom-up approach in which reaction occurs with the help of reducing and stabilizing agents. Bisphosphonates are increasingly being used to treat a wide range of skeletal issues, including hereditary skeletal disorders in children, cancer-related bone metastases and osteoporosis. Asparagus racemosus is a green edible medical plant used in Ayurvedic preparations to treat aging, vigor, immunity, longevity, and skeletal issues. It has been shown to have a variety of pharmacological properties, including antioxidant, antimicrobial, anti-inflammatory, and antiseptic properties. Bisphosphonate conjugated with gold nanoparticle have shown to produce more targeted action. The goal of this study was to green synthesise Bisphosphonate conjugated gold nanoparticles with asparagus racemosus extract and to check their antimicrobial activity and cytotoxicity. Green synthesis of Bisphosphonate conjugated gold nanoparticles using Asparagus racemosus extract was achieved and is characterized. The present study revealed that green preparation of gold nanoparticles is achievable in a simple and eco-friendly manner using Asparagus racemosus root extract. The gold nanoparticles were assessed for their Antibacterial activity against S. mutans, Lactobacillus, and C. albicans. Cytotoxicity of the nanoparticles was assessed using Brine Shrimp Lethality Assay. Gold nanoparticles have good antibacterial activity against Streptococcus mutants (150 ugs/ml–17 mm zone of Inhibition) & Lactobacillus (150 ugs/ml–20 mm zone of Inhibition) and showed 20% lethality at 50ul concentration.
Keywords
Bisphosphonate, Green synthesis, Gold nanoparticles
Introduction
Nanotechnology aims to design, create and control matter in the dimensional range of 1-100nm [1]. The use of materials in these dimensions provides an opportunity to modify various properties such as solubility, diffusivity, blood circulation half-life, drug release characteristics, and immunogenicity at the level of atomic or biomolecules [2,3]. Biological membranes in the human body are selectively permeable to Nanoparticles. The nanoparticles can be surface modified to attach a variety of ligands which enable them to be biosensors, molecular-scale fluorescent tags, imaging agents, and targeted drug delivery vehicles [4-7].
Nanoparticle synthesis by the top-down and bottom-up methods have been described in the literature [8-11]. In general, both methods of nanoparticles use high radiation or concentrated reductants and stabilizing agents that are harmful both to the environment and to human health. Green synthesis of nanoparticles is a type of bottom-up approach in which reaction occurs with the help of reducing and stabilizing agents which eliminates the use of expensive chemicals, consumes less energy, and generates environmentally benign products and byproducts. They have also shown to reduce the toxicity of the nanoparticles. Hence nanoparticles synthesized by green synthesis are considered far more superior to those manufactured through other methods [12-15].
Bisphosphonates are increasingly being used to treat a wide range of skeletal tissues, including hereditary skeletal disorders in children, postmenopausal and glucocorticoid-induced osteoporosis (GIO), and cancerrelated bone metastases, osteoporosis (juvenile, postmenopausal or involutional [senile], glucocorticoidinduced, transplant-induced, immobility-induced, and androgen-deprivation–related), Paget disease of bone, osteogenesis imperfecta (OI), hypercalcemia, and metastatic malignancy. Bisphosphonates can provide significant clinical benefit in diseases characterized by an imbalance between osteoblast-mediated bone formation and osteoclast-mediated bone resorption by destroying the osteoclasts by promoting apoptosis; As a result, bisphosphonates have emerged as an effective treatment for osteoclast-mediated bone resorption. Localized Bisphosphonates have been shown to enhance orthodontic anchorage in several studies [16-19].
Various nanoparticles have shown promise in the regeneration of bone tissue. Gold nanoparticles (GNPs) are a popular choice among these nanoparticles because they are effective at both promoting osteo-differentiation and inhibiting the formation of osteoclasts. GNPs have several advantages, including the ability to accelerate osteoblast differentiation, inhibit adipose-derived stem cell differentiation, suppress osteoclast formation, and promote bone formation in bone tissue regeneration. GNPs have been reported to significantly increase bone tissue regeneration in rabbits .When injected into the body, however, GNPs can cause toxicity. As a result, the surface of these particles must be modified to specifically target bone tissue [20-26].
Asparagus racemosus extracts have previously been used to produce biogenic nanoparticles such as silver, copper oxide, platinum, palladium, and other metals. These nanoparticles have been shown to have antibacterial and cytotoxic properties against a variety of bacteria and cancer cells. Asparagus racemosus (A. racemosus, Shatavari) is a green edible medical plant used in Ayurvedic preparations to treat aging, vigour, immunity, longevity, and skeletal issues. It has been shown to have a variety of pharmacological properties, including antioxidant, antimicrobial, anti-inflammatory, and antiseptic properties. Bisphosphonate conjugated with gold nanoparticles has been shown to provide a more targeted action [27] . The goal of this study was to green synthesize Bisphosphonate conjugated gold nanoparticles with cissus quadrangular is extract and to study their antimicrobial and cytotoxic properties.
Materials and Methods
Green synthesis gold nanoparticles and conjugation of bisphosphonate
Roots of Asparagus racemosus were dried in an oven at 30°C and ground to a coarse powder. 1gm of available Asparagus racemosus stem powder was boiled at 100 degrees c with 100 ml of distilled water in a beaker. The extract was then filtered using a filter paper to obtain 75ml. To reduce the Au2+ ions, 30 mL of the extract was added to a reaction vessel containing 70 ml of chloroauric acid solution. The nanoparticles were then centrifuged at 1500 rpm for 10 minutes before being redisposed in 20 mL of distilled water. 2mg/ml of Zoledronic acid was added to one part of the sample gold nanoparticle extract and left to stir in a magnetic stirrer overnight.
Antimicrobial activity
Biosynthesized nanoparticles are used in a wide range of biomedical applications. Membrane damage is one of the most common causes of nanoparticle antibacterial properties. Using the agar well diffusion method, the green synthesized bisphosphonate conjugated gold nanoparticles were tested against common oral pathogens such as Candida albicans, Enterococcus fecalis, Staphylococcus aureus, and Lactobacillus. The test organisms (S. mutans and Lactobacillus) were grown in nutrient broth and kept on agar slants for the study. Candida albicans were grown on Rose Bengal agar, which is a yeast-specific medium. Using a sterile cotton swab, the freshly cultured strains were grown and uniformly spread over petri dishes containing MHS agar (Mueller Hinton 2 agar+5% sheep blood). With the help of a steel borer, agar wells measuring 6.0 mm in diameter were punched into the culture plate containing the test microorganisms 35.
A micropipette was used to fill the agar wells with 20μL of different concentrations of nanoparticles (50,100,150g/ ml). As a positive control, 20μL of standard antibiotics (Ampicillin) were used. The diameter of the inhibition zone was measured in millimeters after a 24-hour incubation period at 37°C (mm). All of the tests were performed three times.
Cytotoxicity
The eggs of brine shrimp are purchased to perform the cytotoxic assay on brine shrimp. The eggs are then kept at a temperature of 28°C. Artificial seawater and a 37°C light source are used to hatch eggs. This method was tested in 15 well plates (Figure 1). The newly hatched Nauplii are selected and transferred to each well using a Pasteur pipette. The Gold nanoparticles with and without Bisphosphonate conjugation were introduced into each of the wells of varying concentrations of 5,10,15,25 μL is added to each well, and the volume is adjusted. After 24 hours, the brine shrimp are removed from the 15 well plates and counted with a magnifying glass. After a 24- hour incubation period, the percentage of dead shrimp in each well is calculated.
The number of motile nauplii was calculated to assess the cytotoxicity of the nanoparticles.
Viability was calculated per well by
Viability (in %)=live brine shrimp after exposure/live brine shrimp before exposure X 100%
Result and Discussion
The color change of the reaction mixtures from light yellow to yellow, dark-purple, and dark brown, respectively, could indicate the biosynthesis of Au nanoparticles in the current experiment. In the current experiment, the visual color change from yellow to dark purple was formed in 6 hours. The reduction confirmation of Au++ to Au0, as indicated by the solution's color changing from light brown to dark brown. The brown color variation indicates an incomplete reduction of less concentration in the plant extract solution, whereas the formation of dark brown color at high plant extract concentrations revealed a complete reduction reaction.
In the presence of incident photons, Au nanoparticles displayed the surface plasmon resonance (SPR) band as a result of the metal's conduction and free band electrons collectively oscillating. The intensity of the SPR band is primarily determined by the nature of the nanoparticles used in the synthesis, as well as their composition Furthermore, UV-vis spectroscopy is a key tool for determining the nature of synthesized Au. The analysis was carried out every one hour to determine the changes. The analysis showed a consistent peak after 1 hr of preparation at 540 was constantly observed after 2 hours of the preparation of the sample (Figure 1a and 1b).
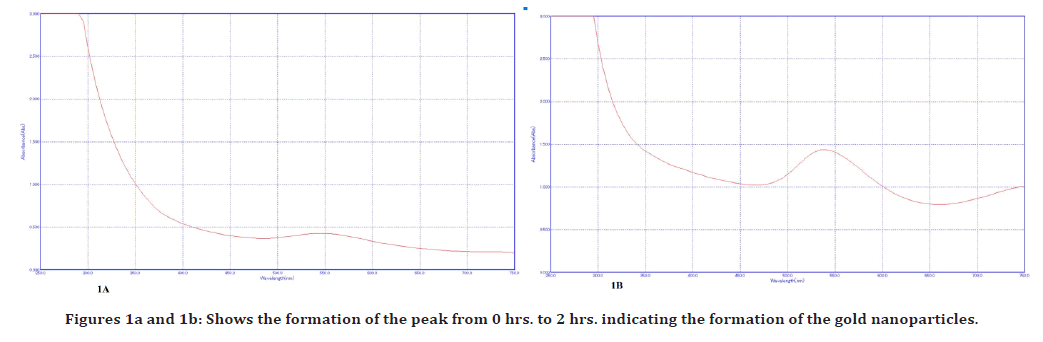
Figures 1a and 1b: Shows the formation of the peak from 0 hrs. to 2 hrs. indicating the formation of the gold nanoparticles.
The TEM images and EDX spectra of biosynthesized Au nanoparticles showed that the particles are narrow in size and spherical in shape with a diameter in the range of 10–50 nm. However, some froth was noticed on the surface of these obtained nanoparticles, which could be attributed to the different types of phytochemicals present in the plant extract. FTIR analysis confirmed the presence of a huge amount of phytochemicals in the plant extract which can prevent the nanoparticles from agglomeration and helps in the production of stable nanoparticles. There was no other defined morphological difference observed in the preparation of Au nanoparticles.
Antibacterial activity of biosynthesized bisphosphonate conjugated gold nanoparticle using Asparagus racemosus extract on Staphylococcus aureus, Lactobacillus, and Candida albicans using agar well diffusion method was performed. This method is widely used to evaluate the antimicrobial activity of plants or microbial extracts. It is qualitative, easy to perform, and simple. The agar plate surface was inoculated by spreading a volume of the microbial inoculum over the entire agar surface. Then, a hole with a diameter of 6 mm was punched aseptically with a sterile corn borer or a tip, and a volume of 20 μL of the nanoparticle sample at desired concentration was introduced into the well. Then, agar plates were incubated under suitable conditions depending upon the test microorganisms. The nanoparticle sample diffuses in the agar medium and inhibits the growth of the microbial strain tested.
In this study, four different concentrations of the nanoparticles were studied (25, 50, 100, 150μg/ml). The diameter of the zone of inhibition increased with an increase in the concentration of the nanoparticles, against S. aureus, Lactobacillus, and Candida albicans. Whereas, the diameter of the zone of inhibition against Enterococcus fecalis showed no change with the concentration of the nanoparticles. The zone of inhibition (in millimeters) of gold nanoparticles of varying concentrations, against S. aureus, Lactobacillus, and Candida albicans are represented in Table 1. Au nanoparticles have good antibacterial activity against Streptococcus mutans (150ug/ml–26 mm zone of Inhibition), Lactobacillus (150 μg/ml–26 mm zone of Inhibition), and Candida albicans (150ug/ml–10 mm zone of Inhibition).
Nanoparticles | Organisms & zone of inhibition for varying concentrations of nanoparticles (ZOI) in millimeter (mm) | ||||||||||||||
---|---|---|---|---|---|---|---|---|---|---|---|---|---|---|---|
Staphylococcus aureus | Lactobacillus | Candida albicans | |||||||||||||
Bisphosphonate conjugated gold nanoparticles | 25 μg/ml | 50 μg/ml | 100μg/ml | 150μg/ml | control | 25μg/ml | 50 μg/ml | 100μg/ml | 150μg/ml | control | 25 μg/ml | 50 μg/ml | 100μg/ml | 150μg/ml | control |
9 | 9 | 9 | 26 | 24 | 9 | 9 | 9 | 26 | 26 | 9 | 9 | 9 | 10 | 12 |
Table 1: Antimicrobial activity of Bisphosphonate conjugated gold nanoparticles at various concentrations.
Cytotoxicity of the prepared nanoparticles was assessed using Brine Shrimp (Artemia salina) Lethality Assay. It has been demonstrated that the early developmental stages of Artemia salina are highly vulnerable to toxins. The lethality was found to be directly proportional to the concentration of the nanoparticles. Gold nanoparticles both with and without Bisphosphonate conjugation showed mortality of 10% at 20 and 25 μg/ml (Table 2).
Concentration (μg/mL) | No. of live Nauplii (Day 1) | No. of live Nauplii (Day 2) | % DEAD |
---|---|---|---|
Control | 10 | 10 | 0 |
Gold nanoparticles without bio phosphate | |||
5 μg/ml | 10 | 10 | 0 |
10 μg/ml | 10 | 10 | 0 |
15 μg/ml | 10 | 10 | 0 |
20 μg/ml | 10 | 9 | 10% |
25 μg/ml | 10 | 9 | 10% |
Gold nanoparticles with bio phosphate | |||
5 μg/ml | 10 | 10 | 0 |
10 μg/ml | 10 | 10 | 0 |
15 μg/ml | 10 | 10 | 0 |
20 μg/ml | 10 | 8 | 20% |
25 μg/ml | 10 | 9 | 10% |
Table 2: Calculation of cytotoxicity at various concentrations of nanoparticles.
Conclusion
We report the Green synthesis and characterization of bisphosphonate conjugated gold nanoparticles with Asparagus racemosus root extract for potential application in Dental Biology.
Funding
Nil.
Informed Consent Statement
Not applicable.
Data Availability Statement
Data available on request.
Conflict of Interest
There are no conflicts of interest.
References
- Zhang L, Gu FX, Chan JM, et al. Nanoparticles in medicine: Therapeutic applications and developments. Clin Pharmacol Ther 2008; 83:761-769.
- Brannon-Peppas L, Blanchette JO. Nanoparticle and targeted systems for cancer therapy. Adv Drug Deliv Rev 2004; 56:1649-1659.
- Kawasaki ES, Player A. Nanotechnology, nanomedicine, and the development of new, effective therapies for cancer. Nanomed Nanotechnol Biol Med 2005; 1:101-109.
- Longmire M, Choyke PL, Kobayashi H. Clearance properties of nano-sized particles and molecules as imaging agents: Considerations and caveats. Nanomed 2008; 3:703–717.
- Chatterjee DK, Fong LS, Zhang Y. Nanoparticles in photodynamic therapy: An emerging paradigm. Adv Drug Deliv Rev 2008; 60:1627–1637.
- Agarwal H, Kumar SV, Rajeshkumar S. A review on green synthesis of zinc oxide nanoparticles–An eco-friendly approach. Resource-Efficient Technol 2017; 3:406-413.
- Rajeshkumar S, Naik P. Synthesis and biomedical applications of Cerium oxide nanoparticles-A review. Biotechnol Rep 2018; 17:1–5.
- https://www.worldscientific.com/worldscibooks/10.1142/7885#t=aboutBook
- Gerberich WW, Jungk JM, Mook WM. The bottom-up approach to materials by design. In Nano and Microstructural Design of Advanced Materials. Elsevier Science Ltd. 2003; 211-220.
- Mohanpuria P, Rana NK, Yadav SK. Biosynthesis of nanoparticles: Technological concepts and future applications. J Nanopart Res 2008; 10:507-517.
- Luechinger NA, Grass RN, Athanassiou EK, et al. Bottom-up fabrication of metal/metal nanocomposites from nanoparticles of immiscible metals. Chem Mater 2010; 22:155-160.
- Anastas PT, Warner JC. Green chemistry: Theory and practice. Oxford University Press, USA 2000; 135.
- Kharissova OV, Dias HR, Kharisov BI, et al. The greener synthesis of nanoparticles. Trends Biotechnol 2013; 31:240-248.
- Narayanan KB, Sakthivel N. Green synthesis of biogenic metal nanoparticles by terrestrial and aquatic phototrophic and heterotrophic eukaryotes and biocompatible agents. Adv Colloid Interface Sci 2011; 169:59-79.
- Singh M, Manikandan S, Kumaraguru AK. Nanoparticles: A new technology with wide applications. Res J Nanosci Nanotechnol 2011; 1:1-1.
- Nakaš E, Lauc T, Tiro A, et al. Dose-and time-dependent effects of clodronate on orthodontic tooth movement. Bosnian J Basic Med Sci 2017; 17:23.
- Fernández‐González FJ, López‐Caballo JL, Cañigral A, et al. Osteoprotegerin and zoledronate bone effects during orthodontic tooth movement. Orthod Craniofac Res 2016; 19:54-64.
- Seifi M, Asefi S, Hatamifard G, et al. Effect of local injection of Zolena, zoledronic acid made in Iran, on orthodontic tooth movement and root and bone resorption in rats. J Dent Res Dent Clin Dent Prospects 2017; 11:257–264.
- Putranto R, Oba Y, Kaneko K, et al. Effects of bisphosphonates on root resorption and cytokine expression during experimental tooth movement in rats. Orthod Waves 2008; 67:141-149.
- Santhoshkumar J, Rajeshkumar S, Kumar SV. Phyto-assisted synthesis, characterization and applications of gold nanoparticles–A review. Biochem Biophys Report 2017; 11:46-57.
- Kattumuri V. Gold nanoparticles for biomedical applications: Synthesis, characterization, in vitro and in vivo (Doctoral dissertation, University of Missouri--Columbia).
- Noah N. Green synthesis: Characterization and application of silver and gold nanoparticles. In Green synthesis, characterization and applications of nanoparticles. Elsevier 2019; 111-135.
- Shukla AK, Iravani S. Green synthesis, characterization and applications of nanoparticles. Elsevier 2018; 548.
- Dauthal P, Mukhopadhyay M. Phyto-synthesis and structural characterization of catalytically active gold nanoparticles biosynthesized using Delonix regia leaf extract. Biotech 2016; 6.
- Kus-Liśkiewicz M, Fickers P, Ben Tahar I. Biocompatibility and cytotoxicity of gold nanoparticles: Recent advances in methodologies and regulations. Int J Mol Sci 2021; 22.
- Fan L, Wang W, Wang Z, et al. Gold nanoparticles enhance antibody effect through direct cancer cell cytotoxicity by differential regulation of phagocytosis. Nat Commun 2021; 12:6371.
- Lee D, Heo DN, Kim HJ, et al. Inhibition of osteoclast differentiation and bone resorption by bisphosphonate-conjugated gold nanoparticles. Sci Rep 2016; 6:27336.
Google Scholar, Indexed at, Cross Ref
Google Scholar, Indexed at, Cross Ref
Google Scholar, Indexed at, Cross Ref
Google Scholar, Indexed at, Cross Ref
Google Scholar, Indexed at, Cross Ref
Google Scholar, Indexed at, Cross Ref
Google Scholar, Indexed at, Cross Ref
Google Scholar, Indexed at, Cross Ref
Google Scholar, Indexed at, Cross Ref
Google Scholar, Indexed at, Cross Ref
Google Scholar, Indexed at, Cross Ref
Google Scholar, Indexed at, Cross Ref
Google Scholar, Indexed at, Cross Ref
Google Scholar, Indexed at, Cross Ref
Google Scholar, Indexed at, Cross Ref
Google Scholar, Indexed at, Cross Ref
Google Scholar, Indexed at, Cross Ref
Google Scholar, Indexed at, Cross Ref
Google Scholar, Indexed at, Cross Ref
Google Scholar, Indexed at, Cross Ref
Google Scholar, Indexed at, Cross Ref
Google Scholar, Indexed at, Cross Ref
Google Scholar, Indexed at, Cross Ref
Author Info
Sruthi H1*, Navaneethan R1 and Rajeshkumar S2
1Department of Orthodontics and Dentofacial Orthopaedics, Saveetha Dental College and Hospital Saveetha Institute of Medical and Technical sciences (SIMATS), Saveetha University, Chennai, India2Department of Pharmacology, Saveetha Dental College and Hospital, Saveetha Institute of Medical and Technical Sciences (SIMATS), Saveetha University, Chennai, India
Received: 18-Jul-2022, Manuscript No. jrmds-22-69406; , Pre QC No. jrmds-22-69406(PQ); Editor assigned: 20-Jul-2022, Pre QC No. jrmds-22-69406(PQ); Reviewed: 04-Aug-2022, QC No. jrmds-22-69406 (Q); Revised: 09-Aug-2022, Manuscript No. jrmds-22-69406 (R); Published: 16-Aug-2022