Research - (2022) Future Prospects of clinical and Medical Research
Challenges in Development of Vaccines for Virus
Dinesh Kumar Yadav1*, Rinka Juneja2, Priyank Bharati3 and Khushboo4
*Correspondence: Dinesh Kumar Yadav, Department of Pharmacognosy, SGT University, Gurugram, Haryana, India, Email:
Abstract
The very advanced avoidance mechanisms of diseases for which vaccines aren’t yet accessible make human vaccine generation dfϔcultǤ Novel vaccine strategy has seen both successes and failures in recent years, and the value of iterative techniques is becoming more widely recognized. To speed vaccine design, these integrate preclinical discoveries of innovative antigens, adjuvants, & plasmids with computer assessments of clinical data. Bio-signatures based on gene transcription proϔles & immunological constraints in patients, vaccinations, & healthy volunteers have been developed to provide suggestions for future vaccine formulation. Public mandate for safe & efϔccous vaccines endures to be strong. In spite of this requirement, only 4 of the big pharmaceutical ϔrms have the expertise & amenities needed to design & produce a new vaccine candidate, in disparity to pharmaceuticals or therapeutic peptides. The current study reviews the challenges in the development of vaccine with special emphasis on human papilloma virus and rota virus.
Keywords
Challenges, Human papilloma virus, Rota virus, Vaccines
Introduction
Vaccines are often regarded as one of medicine's most stunning success stories. They have significantly abridged the beleaguered infectious illness in all known cases. The capacity of currently existing vaccinations to produce antibodies that wedge or kill infectious pathogens or their products is the key to their success. In some infectious illnesses, antibody-mediated protection is insufficient. Antibodies and cell-mediated immunity both have a role in limiting the spread of today's leading infectious killers for which we do not yet have vaccinations. For new vaccines against infectious diseases, there is presently a significant clinical advancement pipeline. After proving protective effectiveness in animal models or a human contest model in the instance of malaria & other disorders, these candidates have been allowed into clinical trials. They were created, however, without any understanding of the relationships between vaccine-induced immunity,biomarkers, and a biological signature that can forecast lower disease recurrence. In theory, clinical trials should generate enough information to allow the creation of a bio-signature which can be utilized to forecast vaccine safety & effectiveness in fresh candidates.
The most challenging technical challenge is scaling up and consistently running a vaccine-making system established in a basic research center into a procedure that can be amplified & operated in a generating environment to generate numerous lakhs of doses per annum. Numerous university & government investigation institutions, as well as start-up biotechnology companies, have the capacity to innovate & create novel vaccine candidates that is the first & most crucial stage in the vaccine creation phase. This is only the initiation of a round of intensive action that will need a high degree of skill, sophisticated equipment, persistence, & patience.
R&D challenges include reducing the time between vaccine candidate discovery, production, and clinical development, making sure that native antigens are reproduced correctly in vaccines, and ensuring that the immune reply triggered is strong enough for effective protection, as well as forecasting at early phases safety plus effectiveness of vaccine candidates.
Vaccine target discovery
The ability to identify vaccine targets has been greatly extended because to the sequencing of entire microbial genomes. The 'reverse vaccinology' technique initiated with the MenB genome, for which traditional methods had very little success, and focused on proteins that were expected to be surface uncovered or discharged. Nominee antigens were selected depending upon their potential to produce bactericidal antibodies that have been related to disease fortification in the past. This method reduced the time it required to identify vaccination targets from decades to a few years, allowing the screening of hitherto undiscovered antigens. 3 antigens with outer membrane vesicles were shown to have a high safety profile, robust immunogenicity, & the capability to cover the bulk of MenB strains universally in clinical studies in grownups, adolescents, & youngsters. The European Medicines Agency approved the first MenB vaccination (commercial name: Bexsero) in 2012 based on the findings of these research.
Reverse vaccinology is currently used to treat a wide range of different infections. The employment of genetics, proteomics, bioinformatics & protein arrays in tandem can speed up the identification of vaccine targets & succeeding vaccine generation [1], [2].
Challenges in preparation of vaccines
The factor H binding protein (FHbp), a main component of the MenB vaccination, elicits substantial protective antibodies. However, there are over 500 amino acid variations in this antigen that don’t generate crossprotective defense. The idyllic candidate antigen would be capable of eliciting defensive antibodies against all of the FHbp allelic variations. Protective epitopes were discovered in each of the variations after the antigen's 3D structure was elucidated. The identified epitopes were designed to be in the right conformation in chimeric molecules. Many of these generated broad-spectrum protective antibodies, and one was chosen for further investigation.
Vaccines against viruses are being rationally designed using structure-based information. The assembly of the F protein of the Respiratory Syncytial Virus allows for the creation of a new F protein with a stable protective antigenic location. The consolidated neutralizing portion of the F protein was used to immunize mice and monkeys, resulting in high quantities of protective antibodies. Similarly, cryo-electron microscopy provided new data on the trimer construction, linkages among gp140 and gp41, & associations with the CD4 interaction site & mitigating antibodies in the HIV gp140 trimer crosslinked through a widely antagonistic antibody. This opens the door to the generation of HIV vaccines founded on structure [3]–[5].
Purified proteins are not immunogenic and must be combined with adjuvants to elicit efficient responses. TLR inhibitors have been approved for usage in a human papillomavirus vaccination. Dispersions with a recyclable lipid core are an example of another adjuvant. Seasonal & pandemic influenza vaccinations (MF59) & pandemic influenza vaccines (AS03) have been approved for use (AS03). These adjuvants alter the quality of the antibody reply in respect of both the width of strains recognized & the repertory of B-cell epitopes, as demonstrated by MF59 with H5N1 & periodic subunit vaccines. The MF59 adjuvant improves the efficiency and efficiency of MF59- adjuvanted vaccinations in newborns & the elderly by eliciting antibody replies to the HA1 component of hemagglutinin (HA), particularly to the binding domain, rather than the HA2 moiety. These events may explain why MF59-adjuvanted vaccinations have a higher effectiveness in babies & the elderly [6], [7].
Vaccines versus an influenza outbreak should be accessible before the breakout. During the last H1N1 pandemic, widespread vaccination wasn't started until transmission had slowed. A fast response to an outbreak is now feasible owing to in vitro genome creation. As a prerequisite, genomes of the viruses obtained must be disclosed as quickly as they become accessible. A mixture of enzymatic, cell-free module methods coupled with enzymatic fault rectification enables for the fast & precise production of genes that would be used to transfect vaccine-qualified cell lines & subsequently salvaged for vaccine production. It may take as little as five days to recover artificial viruses that become antigenically similar to wild-type viruses [6].
Referring to Figure 1, repetition of rudimentary research, vaccine testing, medical study of usual infection, & displaying by computational examination make up the ‘life cycle' of present vaccine designing. Vaccine construction for HIV/AIDS, malaria, & tuberculosis (TB) begins with fundamental research that establishes evidence of concept for a new vaccine candidate. Valid candidates are enrolled in clinical trials that will provide not just evidence regarding security & effectiveness, but also bio-signatures of interest. Clinical research on naturally infected and diseased people, on the other hand, can reveal disease-related bio-signatures. New hypotheses will be generated by computational analysis and modeling, which will be validated by basic research. This iteration could result in a better vaccination [8].

Figure 1: Life cycle of modern vaccine development.
The intricacy of the expertise, the necessity for affluent specialist amenities & the stringent supervisory supplies all provide daunting obstacles for a new entrant into this field. The most difficult challenge is turning basic science advancements into practical vaccinations that can be produced on a large enough scale to have a significant communal health influence while adhering to tight regulatory criteria. The production of millions of doses per annum is vital for a vaccination with widespread utility. The overall quality goal is for all doses to be equal, harmless, & effective. The compliance burden is the quantity of evidence & paperwork needed to demonstrate that this broad quality objective is consistently fulfilled. The most urgent technical challenge is converting a vaccine-making technology created in a rudimentary research laboratory into a procedure that can be amplified & operated consistently in a manufacturing environment to generate millions of doses annually. Many university & government research institutions, along with start-up biotechnology companies, have the capacity to develop & create new vaccine formulation candidates that is the first & most crucial step in the vaccine creation phase. However, this is only the start of a series of powerful action that will necessitate a high level of talent, complex equipment, perseverance, and patience.
Regulatory considerations
The complex procedure of technology creation must be carried out under a regulatory framework that is, in many ways, far more restrictive than that which governs the production of therapeutic polypeptides or smallmolecule medicines. The statistic that vaccinations are significantly more challenging to illustrate rationally than many medications is one reason for the increasing rigor. Simply defined, a small-molecule medication has a molecular weight of less than thousand, analytical examination can confirm its identification & composition, and the ultimate product is dosed according to its weight [9].
The Virus-Like Particle (VLP) employed as the foundation for a vaccine versus the human papillomavirus is bigger than a small-molecule medicine, & the dosage is decided by both weight & biological effectiveness. Due to the difficulties of studying such a large molecule, there has long been a propensity in vaccine creation that the process of developing the vaccine specifies the product, culminating in much more regulatory oversight than a small-molecule medication. Additionally, any modifications in size or technique need confirmation that the product's effectiveness & security will not be jeopardized, & major government regulators expect this proof. Such linking for a shift in method or scale might be followed by very complex & comprehensive analytical depiction, as well as the possibility of further medical testing simply to establish compatibility among the older & newer vaccines [10].
Importantly, since vaccines are administered into the bodies of fit youngsters & grownups to avoid illnesses instead of the traditional medication that is employed to cure individuals who are sick, the requirement for vaccine safety is very high. As a consequence, the vaccination risk-benefit ratio properly highlights the need of well-studied and safe immunizations. The rigorous regulatory monitoring that follows has an effect on every stage of the vaccine generation procedure, from cell line screening until ultimate vaccine quality checks. Every stage of the vaccine production process, comprising the kind & performance of the particular apparatus used for each phase, must be defined & characterized in great detail. These requirements demand a level of discipline in the concept that is intolerant of errors. As a result, every blunder along the route might result in a lengthy (often years) and costly correction in the production process [11].
Literature Review
In their research, Petra et al. concluded that vaccine development has played a critical role in the fight against infectious illness. Despite this progress, new vaccinations are still desperately needed, and they are coming more slower than anybody would want. Despite significant progress in our understanding of immune responses to infection, research is often hampered by a lack of knowledge of the immunological responses needed for protection, or a lack of sanctioned adjuvants & delivery procedures to elicit the requisite responses. Furthermore, the financial investment needed to license novel vaccines is considerable, and the most moneymaking markets are often not the greatest in need. The authors of this review addressed several of the challenges that new vaccinations must overcome in order to decrease morbidity and mortality, as well as some of the efforts being made to get new vaccines to people who need them the most[12].
In his article, John R Middleton looked at the antigens targeted by S. aureus vaccines as well as possible explanations for the lack of effectiveness of S. aureus immunization. In people and animals, S. aureus is a usual basis of nosocomial & community-attained contaminations, & mastitis in dairy cattle. Because methicillin-resilient S. aureus is becoming more widely renowned as a source of staphylococcal contagion, immuno-therapeutics are gaining popularity in both human and animal medicine. Many years have been spent researching vaccines to prevent S. aureus infection in people and mastitis in dairy animals. While certain designs have showed potential in improving medical illness, few, if any, S. aureus vaccines have shown to be effective in preventing new infections [13].
Discussion
The challenges faced in development of Human papillomavirus and rota virus are given below.
Human papillomavirus
Some fresh instances from Merck Research Laboratories for 3 vaccination product candidates demonstrate the various obstacles that come with translating a fundamental vaccine concept into an actual vaccine product. These initiatives have been in the works for at least seven years and are well started. In fact, it is expected that they would be licensed in 2005 in the US & numerous other nations.
The first one is a human papillomavirus (HPV) vaccination that defends versus four distinct strains of the virus (HPV). This artifact entrant was permitted via Commonwealth Serum Laboratories (Australia) & the University of Queensland following comprehensive animal investigations employing VLPs—which are generated by a procedure of self of the HPV L1 peptide. These early experiments demonstrated a good preclinical ‘proof of concept,'1 indicating that this technique has the potential to be developed as a human preventive vaccination. VLP was chosen as an antigen for the reason that the product is inherently noninfectious & since VLPbased hepatitis B vaccines have a strong track record2. The preclinical-to-clinical conversion procedure for the HPV vaccination started in 1993 & lasted 9 years to finish. The second crucial step in the procedure was to develop a vaccine for quick clinic assessment (predicated on its potential to create antibodies against the HPV virus), with the insight that the procedure employed to develop the vaccine might be vital & set the tone for all successive stages of the development programme.
An approach was established to formulate the vaccine for first medical trials that was conceptually comparable to one that would be used for full-scale manufacture if the vaccine was licenced. A vital initial step was the creation of a cell-dependent expression technique that was robust across many generations & generated huge numbers of high-quality VLPs. Saccharomyces cerevisiae was selected as the intracellular VLPs expression host as it can readily be propagated in fermentors of quite few 1000 liters & produces intracellular VLPs consistently throughout its development cycle. Merck Research Laboratories' extensive expertise with S. cerevisiae in the creation of a successful hepatitis B vaccine led to the selection of this host cell (Recombivax). The selection of this yeast strain was made early in the program predicated on productivity, reproducibility, & precise fermentation circumstances, enabling procedure advancement to concentrate on scale-up, refinement, design, & the analytical approaches prerequisite for product depiction & pharmacodynamic measurements.
Following the choice of the vaccine expression technique, a chief seed bank was produced from the modified yeast strain, which was big enough to be used throughout the program. Each of the 4 HPV types incorporated in the vaccine possessed VLP, necessitating technology advancement for the fermentation method, cell generation, cell breakdown, cell debris elimination, chromatography-dependent distillation, & assimilation to the adjuvant of selection for every VLP in order to produce the intended end-product.
The aluminum-based adjuvant was selected to complement that of the Recombivax hepatitis B vaccination, or the VLPs may be stored refrigerated for many years. Investigative approaches dependent on antibody linking to the workable epitopes of the VLPs were developed to estimate the vaccine's effectiveness & to track VLP stability over time when stored as a frozen end-product, as well as to individually evaluate each VLP signifying the different HPV types enclosed in the vaccine.
The objective is to find an antibody which identifies the working epitope of the HPV strain in query while avoiding interference from the vaccine's other three HPV strains. Other important tests to look for contaminants were also developed, such as very subtle assays to sense minute levels of unwanted yeast cell proteins. A main test in saleable vaccine production is ensuring that the procedure is ascendable enough to build a factory capable of producing lakhs of doses while also ensuring that vaccine produced at this measure behaves in the clinic in similar way as vaccine produced at a much lesser measure earlier in the programme.
Furthermore, the insights must be well-fabricated enough that the exact explanation of effectiveness at any specific moment in the design cycle doesn’t change with programme progresses. The ability to connect medical performance, contrasting vaccines produced at an early stage to those used for last phase & clinic testing, is critical. In the instance of the HPV vaccination, the vaccine utilized in stage 1 medical trials was generated in a pilot plant utilizing a less-developed form of the production procedure & on a lower scale (300 ltr). The bulk production of stage 3 vaccination was done using full-size production that is within a multiple of ten of pilot-scale production.
So, what are the fundamental constituents for the effective creation of a large-scale production procedure for the HPV vaccine? First and foremost, amenities must generate representative clinical supplies on a trial size. The second step is to design and build a production facility. Historically, the firm that develops the vaccine has erected these facilities. Third, prior to licensing, vaccine stocks must be produced at this facility, & actual doses must typically be evaluated in the clinic as component of the required procedure for vaccine licensure clearance. Fourth, in order to establish a justification for making minor changes to the process or altering the size of manufacturing & linking without the need for medical validation, comprehensive analytical analysis is needed. Fifth, a medical research group must be competent of organizing large, complicated clinical trials & developing surrogate protective serological tests. Sixth, the legislative requirements for filing across the world must be well recognized upfront in the procedure development endeavor.
A dedicated team of 10 or more individuals is needed to manage & organize such an intrinsically complex venture; this team comprise members from the procedure development board, the analytic group, the medical team, production, advertising, & the internal control organization. To finish this cycle, patience and determination are required, which can take up to ten years or more. After all of this work, the final product is a 0.5 ml vaccine in a vessel or syringe that contains pure VLPs of 4 discrete HPV strains at an accurate & repeatable dose for each, as well as adjuvant.
Rotavirus
The rotavirus vaccine that is created is a live weakened virus vaccine which incorporates 5 distinct humanbovine virus candidates that have been scaled up using VERO cells (Figure 2). Rotavirus belongs to the Reovirus genus, which is part of the Reoviridia virus family. The ultimate reassorted strains were picked from all 5 ultimate kinds by utilising the rotavirus genes' natural capacity to reassort in cell culture. In terms of the attitude for producing a vaccine, the broad outline given for HPV would apply, but the technology-linked challenges are considerably different. The development of masterseeds for every one of the 5 rotavirus attendees, as well as the VERO cell bank, is required for the manufacture of rotavirus vaccines. Each reassortant passes through a different phase, for a total of five. After growing the VERO cells to convergence on a surface, they are infected with rotavirus. Oral administration is the preferred method. Potency is determined by quantifying weakened live virus employing PCR technique that is utilized to count each of the discrete 5 reassortants in the ultimate produced vaccine. The medical trials for this vaccine, which were necessary to confirm both security & effectiveness, were extremely big and costly, needing more than 100,000 vaccine doses created using a procedure similar to that used in final-scale manufacturing.
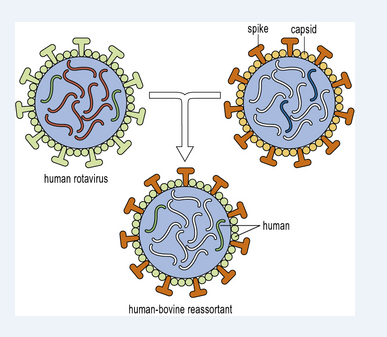
Figure 2: Illustration of development of humanbovine reassortant for development of RotaTeq vaccine.
RotaTeq is a complexing vaccine comprising of 5 humanbovine reassortants: 4 G serotypes (G1, G2, G3, G4) that represent eighty percent of the G strains cycling worldwide, plus one P serotype that represents more than 75 percent of the P strains cycling globally. Figure 3 is an illustration of the timeline of development of RotaTeq vaccine.
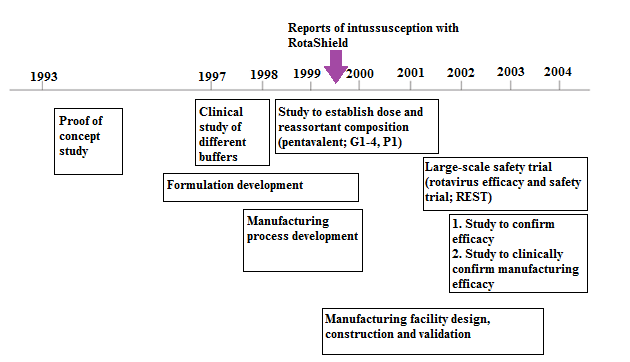
Figure 3: Overview of development of RotaTeq.
Conclusion
In the near future, the vaccine community will face the problem of better balancing the risk-benefit equation depending on the envisioned application. A vaccination envisioned for widespread pediatric use, for example, requires both efficacy evidence and rigorous safety testing. In contrast, if a vaccine is developed in response to a potential bioterrorism threat, it may only be used in an emergency circumstance and efficacy testing in people may not be viable. In the latter case, a more straightforward and expedited approach to approval could be preferable. In the manufacturing of new vaccines, method progress must be coordinated with the creation of improved analytical techniques for vaccine characterisation & potency evaluation. These operations must be properly integrated with a strategy for clinical evaluation, supervisory authorization, & manufacturing. The procedure design scientist or technician must be fully acquainted of all of these linked concerns in order to magnificently deliver a technology viaduct amid an exciting new research run & the production of the finished product setting the groundwork for final licensure & release of a novel vaccine.
Although there is still a long way to go in developing novel vaccines, recent improvements in antigen discovery, adjuvant creation, & vector scheme have accelerated the pre-medical vaccine R&D channel. Such achievements are complimented by current achievements in human vaccinology, such as the lucid design of bio-signatures, which can be used to improve future vaccine candidates by forecasting disease risk and vaccine care & effectiveness. As a result, a repetitive mixture of wet-lab, in silico analysis, & clinical investigations is the best strategy to speed up vaccine development for major infectious illnesses. Even if the initial cohort of vaccine candidates does not show sufficient effectiveness, comparing evaluations of host reactions in sheltered with defenseless research participants will disclose knowledge that will aid in the development of future vaccines. Adoptive vaccine regimens would be best adapted to capture emerging insights resulting from data analysis of ongoing vaccine trials.
References
- Khan AM, Hu Y, Miotto O, et al. Analysis of viral diversity for vaccine target discovery. BMC Med Genet 2017;10(Suppl 4):78.
[Crossref], [GoogleScholar], [Indexed]
- Chong LC, Khan AM. Vaccine target discovery. In Encyclopedia of ABC of Bioinformaticswe2018;3:241-251.
[Crossref], [GoogleScholar], [Indexed]
- Mugyenyi PN. HIV vaccines: The Uganda experience. Vaccine 2002;20(15):1905â??1908.
[Crossref], [GoogleScholar], [Indexed]
- Khalid K, Padda J, Khedr A, et al. HIV and messenger RNA vaccine. Cureus 2021;13(7):e16197.
[Crossref], [GoogleScholar], [Indexed]
- Nadeem MS, Ali A, Al-Ghamdi MA, et al. COVID-19: Prospective challenges and potential vaccines. Altern Ther Health Med 2020. [Crossref],
[GoogleScholar], [Indexed]
- Mcelhaney JE, Andrew MK, Haynes L, et al. Influenza vaccination: Accelerating the process for new vaccine development in older adults. Interdiscip Top Gerontol Geriatr 2020;43:98â??112.
[Crossref], [GoogleScholar], [Indexed]
- Rockman S, Laurie KL, Parkes S, et al. New technologies for influenza vaccines. Microorganisms 2020;8(11).
[Crossref], [GoogleScholar], [Indexed]
- Singh K, Mehta S. The clinical development process for a novel preventive vaccine: An overview. J Postgrad Med 2016;62(1):4â??11.
[Crossref], [GoogleScholar], [Indexed]
- Heelan BT. Regulatory considerations for clinical development of cancer vaccines. Hum Vaccin Immunother 2014;10(11):3409â??3414.
[Crossref], [GoogleScholar], [Indexed]
- Yang S, Fink D, Hulse A, et al. Regulatory considerations in development of vaccines to prevent disease caused by Chikungunya virus. Vaccine 2017;35(37):4851â??4858.
[Crossref], [GoogleScholar], [Indexed]
- Naik R, Peden K. Regulatory considerations on the development of mRNA vaccines. Curr Top Microbiol Immunol 2020:1-19.
[Crossref], [GoogleScholar], [Indexed]
- Oyston P, Robinson K. The current challenges for vaccine development. J Med Microbiol 2012;61(7):889â??894.
[Crossref], [GoogleScholar], [Indexed]
- Middleton JR. Staphylococcus aureus antigens and challenges in vaccine development. Expert Rev Vaccines 2008;7(6):805â??815.
[Crossref], [GoogleScholar], [Indexed]
Author Info
Dinesh Kumar Yadav1*, Rinka Juneja2, Priyank Bharati3 and Khushboo4
1Department of Pharmacognosy, SGT University, Gurugram, Haryana, India2Department of Pharmacy, Sanskriti University, Mathura, Uttar Pradesh, India
3School of Biotechnology & Bioinformatics, Shobhit Institute of Engineering and Technology (Deemed to, India
4Department of Biotechnology, RIMT University, Mandi Gobindgarh, India
Received: 02-May-2022, Manuscript No. JRMDS-22-58458; , Pre QC No. JRMDS-22-58458(PQ); Editor assigned: 04-May-2022, Pre QC No. JRMDS-22-58458(PQ); Reviewed: 16-May-2022, QC No. JRMDS-22-58458; Revised: 18-May-2022, Manuscript No. RMDS-22-58458(R); Published: 25-Jun-2022