Research - (2022) Volume 10, Issue 9
Cytotoxic Effect of Lycopene Mediated Silver Nanoparticles in Fibroblast Cell Line Culture-An In Vitro Study
Bhavana Garapati1*, Sankari Malaiappan1, S Rajeshkumar2 and Karthikeyan Murthykumar1
*Correspondence: Bhavana Garapati, Department of Periodontics, Saveetha Dental College and Hospitals, Saveetha Institute of Medical and Technical sciences (SIMATS), Saveetha University, Chennai, India, Email:
Abstract
Background - Nano-silver (AgNP) has biological properties which are significant for consumer products, food technology, and medical applications (e.g. wound care products, implantable medical devices, in diagnosis, drug delivery, and imaging). Thus, the use of nano-silver is becoming more and more widespread in medicine. In this study, we investigated the cytotoxic effects of AgNPs on fibroblasts cells of humans. Material and Method - The Lycopene capsule of 0.5mg was dissolved and gently grounded with 100 ml distilled water and The silver nanoparticles were synthesized and added to the lycopene substrate to check the cytotoxic effects of lycopene Ag NP on 3T3 cells. Cells were treated with Lycopene Ag NP (10, 20, 30, 50, 100, and 200 μM) for 24 h, and cell viability was evaluated by MTT assay. Data were compared with the control blank group. Result - The overall result indicates that AgNPs can selectively inhibit the cellular mechanism of fibroblasts. They demonstrated that silver nanoparticles of more than 20 nm increased cytotoxicity in human periodontal fibroblasts in a dose- and time-dependent manner. Conclusion - Has no/less effect on the human fibroblast cells. Lycopene mediated AgNPs are safe to healthy cells at a concentration <20 μg/ml.
Keywords
Lycopene, Silver nanoparticles, Cytotoxicity, Cell line culture
Introduction
Periodontitis and dental caries are the two main oral diseases faced by most humans in their day-to-day life [1]. Nanotechnology is a relatively emerging field of science that has found a wide range of applications that range from energy production to industrial production processes to biomedical applications [1,2]. This is due to the use of metal nanoparticles in a variety of fields, including medicine [3,4], chemical sensing, catalysis, and electronics [5,6]. Nanoparticles are those which have a size of 1 to 100 nm and possess unique physical and chemical properties due to their large surface area to volume ratio and smaller size [6,7]. The three main methods of synthesis of nanoparticles are physical, chemical, and biological. More research into low-cost, non-toxic methods of synthesizing nanoparticles from natural products is required.
Silver nanoparticles have piqued the interest of many researchers due to their unique physical and chemical properties, which allow them to be used in a wide range of applications. Studies have shown that AgNPs have piotent antimicrobial properties and antioxidant properties [8–10]. Silver is a transition metal and is used in medicinal systems, it's a less toxic metal and it has been used in many applications due to the several distinctive properties like optical, electrical, catalytic, antimicrobial, and antioxidant activity [11].
Ernest et al. discovered lycopene, a fat-soluble carotenoid, in 1959. It is found in red fruits and vegetables as a natural component [12]. The main sources of lycopene in the human diet are tomatoes and tomato-based products. Lycopene acts as a good antioxidant as well as helps in the prevention of cancer and oral diseases like leukoplakia, lichen planus, and periodontitis. Lycopene is more effective with other antioxidants like vitamin C. Lycopene has also been associated with decrease in oxidative stress because it is inversely related to malondialdehyde (MDA), a marker of oxidative stress.
The effects of nanoscale particles on organisms, on the other hand, have not been thoroughly investigated. There are numerous approaches to performing toxicity tests at the moment, but common and reasonable bioindicators for toxicity evaluations are lacking. Several mechanisms, most notably the production of excess reactive oxygen species, contributed to AgNPs toxicity (ROS). ROS are both physiologically necessary and potentially destructive. ROS may contribute to tissue damage in many pathophysiological conditions and participate in several cellular events, including signal transduction, proliferative response, gene expression, and protein redox regulation [13,14]. High ROS levels indicate oxidative stress and can influence cellular signal transduction pathways, such as proinflammatory signaling pathways, as well as modulate the expression of many genes. ROS production has been shown to play an important role in AgNP-induced apoptosis [20- 22]. Different types of cell death (apoptosis, necrosis, and autophagy) are now known to contribute to the pathophysiology of various human disorders [23].
The conventional physical and chemical manufacture of AgNPs is simple and can yield a huge quantity of material. However, the process is energy-demanding and timeconsuming and is not eco-friendly because of the use of harmful chemicals. As a result, the development of AgNPs with well-controlled size and tailored morphological and physicochemical properties is required, as is the synthesis of AgNPs without the use of hazardous chemicals. Only a few studies have looked into the synthesis of AgNPs using a pure flavonoid reduction of Ag, as well as the effects on the molecular mechanism of toxicity. It would be beneficial to identify bio-indicators of AgNP toxicity in a mammalian system. Previously mouse embryonic fibroblasts (MEFs) were used to study gene ablations [15,16] and were also involved in wound repair and healing. AgNPs have been exploited in cancer therapy. As a result, a thorough examination of the toxicity of AgNPs effects and the molecular mechanisms of toxicity is required. AgNPs have the ability to modulate epigenetic dysregulation, which is involved in gene expression reprogramming, influence the cell cycle, and induce DNA hyper methylation via the p53 or p21 pathways, all of which can affect the epigenomic level.
Recent advances in next-generation sequencing technologies have enabled the use of high-throughput sequencing of DNA and RNA (the latter referred to as RNA-Seq) from bulk or low-input samples [17]. For gene expression profiling of cells and tissues, the technology is now preferred over cDNA microarrays [18,19]. The RNA-Seq method allows for the simultaneous analysis of a large number of genes/targets as well as the identification of treatment mechanisms [20-23]. The transcriptomic changes and cellular responses of NIH3T3 cells treated with AgNPs, on the other hand, have not been studied. For this study, we chose NIH3T3 as a model system in particular. Fibroblasts are ubiquitous mesenchymal cells that play critical roles in wound repair and healing, as well as reservoirs of multipotent progenitors capable of replenishing depleted cell compartments. MEFs are frequently used in research to investigate the physiological effects of selective gene ablations. The findings of this fibroblast study could provide useful information on the potential health risks associated with human exposure to AgNPs, which could help to develop safe practices for the future use of AgNPs. Understanding the mechanisms of AgNPmediated toxicity should also aid in the identification of protective or preventative measures. The question of whether AgNPs can affect different skin cell types— keratinocytes and fibroblasts—during the wound healing process remains unanswered. Therefore, this study aims to assess the cytotoxic effect of lycopene (0.5mg capsule, commercially available) mediated silver nanoparticles in human fibroblast cell line culture.
Materials and Methods
Preparation of lycopene extract
The Lycopene capsule of 0.5mg was dissolved and gently grounded with 100ml distilled water. Grounded lycopene was boiled at 70ºC for 15 min and filtered using Whatman no.1 filter paper. Collected filtrate was used for the bio reduction of silver ions to silver nanoparticles.
Synthesis of silver nanoparticles
To prepare the silver nanoparticles, 90 mL of 1mM silver nitrate solution was prepared in a 250 mL Erlenmeyer flask and added 10 mL of the filtrate of lycopene in the room temperature. The colour change was observed visually and the synthesis of silver nanoparticles at different time intervals (0 min, 10 min, 20 min, 40 min, 1 h, 2 h, 3 h, 4 h and 24 h) was monitored by the UV-vis spectrophotometer of the solution. To study the effect of substrate concentration, 0.1 mm, 1 mm and 2 mm concentration of silver nitrate solution were prepared and added 10 mL of lycopene to silver nitrate solution and examine the silver nanoparticles synthesis in UV-vis spectrophotometer at a different wavelength (350- 550 nm).
Chemicals and antibodies
DMEM medium, 0.25% Trypsin-EDTA solution, sodium bicarbonate solution, bovine serum albumin (BSA), low melting agarose, MTT from Sigma Chemicals Co., St. Louis, USA. fetal bovine serum (FBS) and antibiotic/ antimycotic solution, DMSO were from Himedia, Sodium phosphate monobasic and dibasic, sodium chloride, sodium hydroxide, sodium carbonate, hydrochloric acid and methanol were purchased from Sisco Research Laboratories (SRL) India.
Cell culture reagents
DMEM
Commercially available DMEM contains 7.5% sodium bicarbonate solution. To 500ml of DMEM, 5ml of penicillin/streptomycin solution and 0.5ml of amphotericin B solution was added. Then the medium was sterile filtered (0.22μ) inside the hood. The medium was then dispensed into a sterile container and stored at 4°C.
Growth medium (DMEM with 10% FBS)
10ml of FBS was made up to 100ml using sterile DMEM. It was kept cool and aseptic in a sterile container.
Phosphate buffered saline (PBS; pH 7.4)
0.63 g of sodium phosphate monobasic (NaH2PO4), 0.17 g of sodium phosphate dibasic (Na2HPO4) and 4.5 g of sodium chloride (NaCl) were dissolved in 500 ml of double autoclaved milliQ water. The pH was then adjusted to 7.4 using 1 N HCl and 1 N NaOH, sterile filtered (0.22 μ) and then stored in a sterile container.
Trypsin-EDTA
Trypsin was purchased as 1 x with EDTA (0.5% trypsin, 5.3 mm EDTA sodium salt). (Note: Freeze-thaw process does not affect the enzyme activity. Thawing is done at room temperature).
Cell line maintenance
Human breast cancer cell line was procured from National Centre for Cell Science (NCCS, Pune), India. The cells were grown in T25 culture flasks containing DMEM medium supplemented with 10% FBS. Upon reaching confluence, the cells were detached using Trypsin-EDTA solution.
Passaging of cells
Upon reaching the confluence cell line the cells were passaged as follows. The medium from the culture flask was aspirated. The flask was rinsed with 2ml of PBS and aspirated again quickly. 1.5-ml of trypsin-EDTA solution was added and incubated at 37ºC for about 2 minutes until cells started detaching. As soon as the cells were detached, transfer the trypsinized medium containing cells using a transfer pipette into a 15 ml falcon tube and it was centrifuged at 1000 rpm for 5 min. The medium was carefully aspirated out, and the pipette tip was not placed in the bottom of the tube, where the cells were pelleted. The cells were gently suspended in the DMEM medium with 10% FBS by pipetting up and down 5-8 times gently. From the cell suspension, a drop was placed on the edge of the coverslip of the neubauer hemocytometer. The drop was led to run under the cover slip by capillary action. Care was taken not to force the liquid and the entry of the air bubble was avoided. Then the cells from the E1, E2, E3, E4 and E5 squares were counted under a microscope. The cells were then gently suspended and transferred to sterile culture flasks and the volume of medium was made upto required volume with growth medium per flask
Cell proliferation (MTT) assay
The proliferation of MCF-7 cells was assessed by MTT assay.
Principle
The assay is based on metabolically active cells that convert soluble yellow tetrazolium salts into insoluble purple formazan crystals. Only living cells can absorb tetrazolium salts. The enzyme (mitochondrial dehydrogenase) found in the mitochondria of living cells can convert the tetrazolium salt inside into purple formazan crystals. Then, cells were lysed with 20% SDS solution, releasing formazan crystals. These crystals are dissolved by the DMF present in the solute. The developed color was then determined in an ELISA reader at 620 nm.
Reagents
• MTT [3-(4,5-dimethylthiazol-2-yl) 2,5-diphenyl tetrazolium bromide]: 0.5 mg MTT/ml of serumfree DMEM.
• Solubilization solution: Dimethyl sulfoxide (DMSO)
• Phosphate Buffered Saline (PBS; pH 7.4).
Procedure
A549 and MCF7 cells were plated in 48-well plates at a concentration of 2x104 cells/well 24 h after plating, cells were washed twice with 500 μl of serum-free medium and starved by incubating the cells in medium. Serumfree field for 3 hours at 37ºC. After starvation, cells were treated with different concentrations of compound names for 24 h. At the end of the treatment, the medium of the cells was treated with the control and the name of the compound removed and 200 μl of MTT containing DMEM (0.5 mg/ml) was added to each well. Then, the cells were incubated for 4 h at 37 °C. in a CO2 incubator.
MTT-containing medium was then removed and cells were washed with 1x PBS. The crystals were then dissolved by adding 200 μl of dissolving solution, and the mixture was mixed well by pipetting up and down. Then, the formazan crystals formed were dissolved in dimethyl sulfoxide (200 μl) and incubated in the dark for one hour. Then, the intensity of the developed color was measured at 570 nm using a Micro ELISA plate reader. The percentage of viable cells grown in serum-free medium was used to represent the number of viable cells. Cell viability was shown to be 100% in control medium without any treatment. Cell viability was calculated as follows: cell viability percentage=[A570 nm of treated cells / A570 nm of control cells] x 100.
Morphological study
Based on the MTT trial, we selected the optimal dose. Use phase contrast microscopy to examine changes in cell morphology. In a 6-well plate, 3104 cells were seeded and treated for 24 h with the name of the compound (concentration for MCF7 cells). At the end of incubation, the medium was removed and the cells were washed once with phosphate-buffered saline (PBS pH 7.4). These patches were observed under a phase contrast microscope.
Results
In the present study, there was not much difference in the cell viability among all concentrations. At a minimum concentration of 10 μg/ml, the percentage inhibition was found to be 86.5%. The maximum cytotoxicity of 86.5% was found at the concentration of 10 μg/ml. At a concentration of 200 μg/ml, AgNPs exhibited 83.36% inhibition. Therefore, a concentration less than 20 μg/ml will be effective against diseased cells and is safe to the healthy cells. The cells have also not shown any damage and are alive at lower concentrations (Figures 1-4).
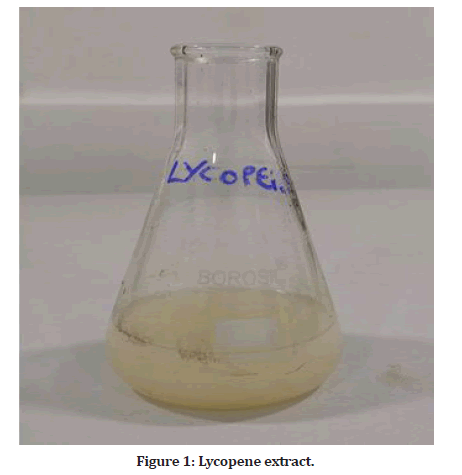
Figure 1: Lycopene extract.
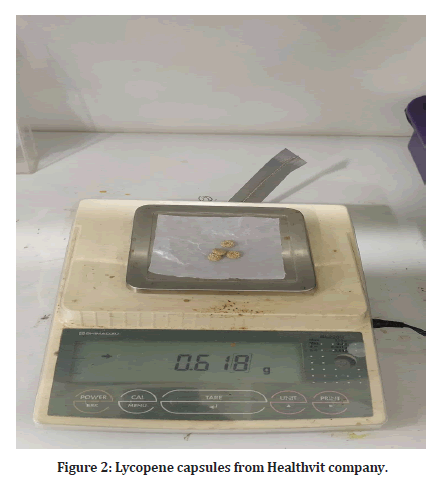
Figure 2: Lycopene capsules from Healthvit company.
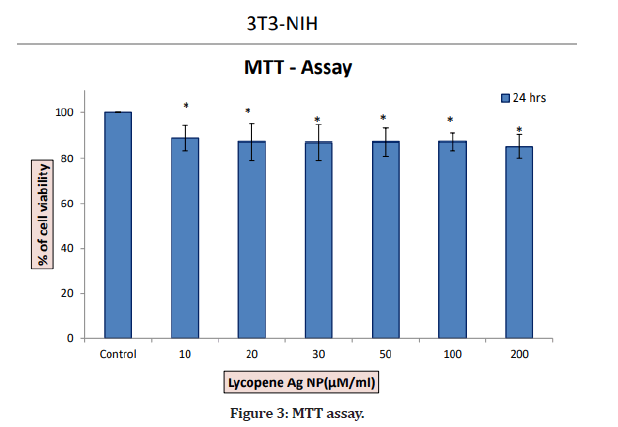
Figure 3: MTT assay.
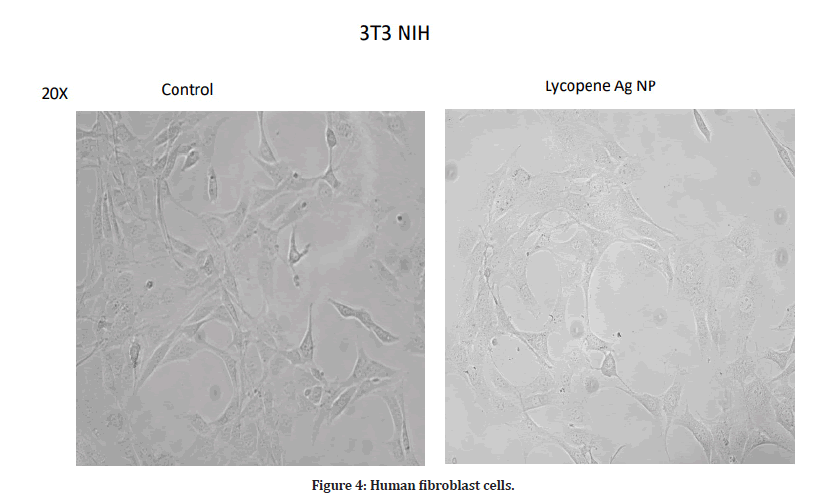
Figure 4: Human fibroblast cells.
Discussion
Recently, there has been an increase in research in synthesizing AgNPs to develop a wide range of applications such as catalysis, biodetection, imaging and antibacterial activity. Green synthesis is an alternative method to create metal nanoparticles using natural compounds or plant components. These are environmentally friendly processes that avoid the toxicity of chemicals. NPs were synthesized using algae, bacteria, fungi and plants without the use of additional reducing agents and stabilizers. The plant extracts contain functional substances including cyclic peptides, asorbic acid, carotenes, retinoic acid, ascorbic acid, and gallic acid, others suspected to play an important role in the process. Bio sorption and stabilization of nanoparticles [24]. The process appears to be easy, safe, inexpensive, and environmentally friendly, eliminating the need to maintain consistent and complicated sterile cell cultures.
Lycopene is useful in the treatment of a number of diseases of the oral cavity including periodontitis, oral cancer and precancerous lesions [25] and its various benefits to human health. Humans can be explained by its properties: 1. Antioxidant activity, 2. Inhibition of the proliferation of cancer cells, 3. Inhibition of growth factor stimulation. 4. Phase II induction of enzymes, 5. Transcriptional regulation and 6. Restoration of gap junctions.
Lycopene exerts its antioxidant activity through physical and chemical quenching of free radicals and is the most essential carotenoid for single oxygen quenching [26]. The unique biochemical properties of Lycopene may make it possible to protect cellular components against specific types of damage induced by highly reactive oxygen species [27]. Therefore, the synthesis of lycopene-mediated silver nanoparticles could have great medicinal value, and they can also be used in various dental treatments. AgNPs can penetrate tissues due to their extremely small size and high surface area, thus potentially being used for drug-resistant bacteria [28]. Nanoparticle cytotoxicity studies are usually performed in vitro because of their ease of implementation, control, and interpretation, and they are preliminary studies that mimic in vivo conditions. Cytotoxicity can be studied in vitro using a number of qualitative and quantitative assays; however, quantitative assays are most appropriate because they quantify the number of viable cells [29].
The MTT assay was chosen for this study because it is the recommended quantitative test for biocompatibility testing is simple to perform, and it allows microscopic analysis to differentiate non-viable cells and viable cells. This is a colorimetric method for the determination of the reduction of yellow MTT by mitochondrial succinate dehydrogenase. Since MTT reduction can only occur in metabolically active cells and cytoreduction can only be catalyzed by living cells, the percentage of viable cells in a solution can be calculated translation [29].
Despite the widespread use of AgNPs in the medical field, relatively few in vitro studies have been performed on healthy cells, most of which have been performed on tumor cells [30]. Different cell lines were used to evaluate cytotoxicity because diploid/primary cells, mainly oral fibroblasts, were considered to be more suitable biological tools for experiments. Compared with permanent cells [31]. However, the literature is sparse on cytotoxicity studies of lycopene-mediated AgNPs in cells such as human fibroblast cells, which are considered common in vitro models for these studies for biocompatibility studies [32] and were therefore used in this study.
In this study, at a minimum concentration of 10 g/ml, the percentage inhibition was found to be 86.5%. A maximum cytotoxicity of 86.5% was found at a concentration of 10 μg/ml. At a concentration of 200 μg/ml, AgNPs exhibited 83.36% inhibition. Therefore, the concentration <= 20 μg/ml will be effective and safe for healthy cells.
Han, et al. [33] reported the cytotoxicity of biosynthesized AgNPs on human lung adenocarcinoma cell lines with an IC50 value of 20 μg/ml compared with the valuable synthetic AgNPs. The IC50 was 70 μg/ml, indicating that AgNPs were biosynthesized very efficiently at minimal doses compared to AgNPs. Since the material of choice used to evaluate cytotoxic effects was completely different and there were no previous studies using the combination of silver lycopene nanoparticles, it was not possible to compare the results as so.
The results of this study indicate that AgNPs are less cytotoxic to the human fibroblast cell line and increase with increasing AgNPs concentration, which is consistent with the study by Magdi, et al. associates [34].
Conclusion
Human fibroblast cells can survive at 10 μg/ml when treated with lycopene-mediated silver nanoparticles. This is the first study in which cytotoxicity has been investigated using lycopene-mediated silver nanoparticles. Further research should be done with different strengths and other combinations in periodontal therapy, as antioxidant properties have been established this combination can be used as a rinse mouth in the future.
References
- Petersen PE, Bourgeois D, Ogawa H, et al. The global burden of oral diseases and risks to oral health. Bull World Health Organ 2005; 83:661â??669.
- Allhoff F, Lin P, Moore D. What is nanotechnology and why does it matter?: From Science to Ethics John Wiley & Sons 2009.
- Rajeshkumar S. Phytochemical constituents of fucoidan (Padina tetrastromatica) and its assisted AgNPs for enhanced antibacterial activity. IET Nanobiotechnol 2017; 11:292â??299.
- Kumar SV, Rajeshkumar S. Optimized production of silver nanoparticles using marine macroalgae Sargassum myriocystum for its antibacterial activity. Jo Bionanosci 2017; 11:323â??329.
- Rajeshkumar S, Malarkodi C, Vanaja M, et al. Anticancer and enhanced antimicrobial activity of biosynthesizd silver nanoparticles against clinical pathogens. J Mol Struct 2016; 1116:165â??173.
- Rajeshkumar S, Malarkodi C. Optimization of Serratia nematodiphila using response surface methodology to silver nanoparticles synthesis for aquatic pathogen control. Mater Sci Eng 2017; 263:022041.
- Agarwal H, Venkat Kumar S, Rajeshkumar S. A review on green synthesis of zinc oxide nanoparticlesâ??An eco-friendly approach. Resour-Effic Technol 2017; 3:406â??413.
- Agarwal H, Menon S, Kumar SV, et al. Mechanistic study on antibacterial action of zinc oxide nanoparticles synthesized using green route. Chem Biol Interactions 2018; 286:60-70.
- Menon S, Ks SD, Santhiya R, et al. Selenium nanoparticles: A potent chemotherapeutic agent and an elucidation of its mechanism. Colloids Surfaces 2018; 170:280-292.
- Kumar SV, Rajeshkumar S. Plant-based synthesis of nanoparticles and their impact. In Nanomaterials in plants, algae, and microorganisms. Academic Press 2018; 33-57.
- Mohanpuria P, Rana NK, Yadav SK. Biosynthesis of nanoparticles: Technological concepts and future applications. J Nanopart Res 2008; 10:507â??517.
- Saawarn N, Saawarn S, Chaitanya N, et al. Lycopene in the management of oral lichen planus: A placebo-controlled study. Indian J Dental Res 2011; 22:639.
- Bedard K, Krause KH. The NOX family of ROS-generating NADPH oxidases: Physiology and pathophysiology. Physiol Rev 2007; 87:245â??313.
- Storz P. Fork head homeo box type O transcription factors in the responses to oxidative stress. Antioxid Redox Signal 2011; 14:593â??605.
- Gurunathan S, Qasim M, Park C, et al. Cytotoxicity and transcriptomic analysis of silver nanoparticles in mouse embryonic fibroblast cells. Int J Mol Sci 2018; 19:3618.
- Singhal PK, Sassi S, Lan L, et al. Mouse embryonic fibroblasts exhibit extensive developmental and phenotypic diversity. Proceedings of the National Academy of Sciences 2016; 113:122â??127.
- Mytych J, Zebrowski J, Lewinska A, et al. Prolonged effects of silver nanoparticles on p53/p21 pathway-mediated proliferation, DNA damage response, and methylation parameters in HT22 hippocampal neuronal cells. Mol Neurobiol 2017; 54:1285â??300.
- Feliu N, Kohonen P, Ji J, et al. Next-generation sequencing reveals low-dose effects of cationic dendrimers in primary human bronchial epithelial cells. ACS Nano 2015; 9:146â??163.
- Liu Y, Guo Y, Ma C, et al. Transcriptome analysis of maize resistance to Fusarium graminearum. BMC Genomics 2016; 17.
- Sun QL, Zhao CP, Wang TY, et al. Expression profile analysis of long non-coding RNA associated with vincristine resistance in colon cancer cells by next-generation sequencing. Gene 2015; 572:79â??86.
- Wang Z, Gerstein M, Snyder M. RNA-Seq: A revolutionary tool for transcriptomics. Nat Rev Genet 2009; 10:57â??63.
- Lin HN, Hsu WL. DART: A fast and accurate RNA-seq mapper with a partitioning strategy. Bioinformatics 2018; 34:190â??197.
- Costa BM, Smith JS, Chen Y, et al. Reversing HOXA9 oncogene activation by PI3K inhibition: Epigenetic mechanism and prognostic significance in human glioblastoma. Cancer Res 2010; 70:453â??462.
- Rajan R, Chandran K, Harper SL, et al. Plant extract synthesized silver nanoparticles: An ongoing source of novel biocompatible materials. Ind Crops Prod 2015; 70:356â??373.
- Lu R, Dan H, Wu R, et al. Lycopene: Features and potential significance in the oral cancer and precancerous lesions. J Oral Pathol Med2011; 40:361-368.
- Stahl W, Sies H. Lycopene: A biologically important carotenoid for humans? Arch Biochem Biophys 1996; 336:1â??9.
- Giovannucci E. Tomatoes, tomato-based products, lycopene, and cancer: review of the epidemiologic literature. J Natl Cancer Inst 1999; 91:317-331.
- Stone V, Johnston H, Schins RPF. Development of in vitro systems for nanotoxicology: Methodological considerations. Crit Rev Toxicol 2009; 39:613â??626.
- Hanks CT, Wataha JC, Sun Z. In vitro models of biocompatibility: A review. Dent Mater 1996; 12:186-193.
- Vivek R, Thangam R, Muthuchelian K, et al. Green biosynthesis of silver nanoparticles from Annona squamosa leaf extract and its in vitro cytotoxic effect on MCF-7 cells. Process Biochem 2012; 47:2405â??410.
- Hauman CHJ, Love RM. Biocompatibility of dental materials used in contemporary endodontic therapy: A review. Part 1. Intracanal drugs and substances. Int Endod J 2003; 36:75â??85.
- Chiang SL, Jiang SS, Wang YJ, et al. Characterization of arecoline-induced effects on cytotoxicity in normal human gingival fibroblasts by global gene expression profiling. Toxicol Sci 2007; 100:66-74.
- Han JW, Gurunathan S, Jeong JK, et al. Oxidative stress mediated cytotoxicity of biologically synthesized silver nanoparticles in human lung epithelial adenocarcinoma cell line. Nanoscale Res Lett 2014; 9:459.
- Magdi HM, Mourad MHE, El-Aziz MMA. Biosynthesis of silver nanoparticles using fungi and biological evaluation of mycosynthesized silver nanoparticles. Egypt J Exp Biol 2014; 10:1â??12.
Indexed at, Google Scholar, Cross Ref
Indexed at, Google Scholar, Cross Ref
Indexed at, Google Scholar, Cross Ref
Indexed at, Google Scholar, Cross Ref
Indexed at, Google Scholar, Cross Ref
Indexed at, Google Scholar, Cross Ref
Indexed at, Google Scholar, Cross Ref
Indexed at, Google Scholar, Cross Ref
Indexed at, Google Scholar, Cross Ref
Indexed at, Google Scholar, Cross Ref
Indexed at, Google Scholar, Cross Ref
Indexed at, Google Scholar, Cross Ref
Indexed at, Google Scholar, Cross Ref
Indexed at, Google Scholar, Cross Ref
Indexed at, Google Scholar, Cross Ref
Indexed at, Google Scholar, Cross Ref
Indexed at, Google Scholar, Cross Ref
Indexed at, Google Scholar, Cross Ref
Indexed at, Google Scholar, Cross Ref
Indexed at, Google Scholar, Cross Ref
Indexed at, Google Scholar, Cross Ref
Indexed at, Google Scholar, Cross Ref
Indexed at, Google Scholar, Cross Ref
Indexed at, Google Scholar, Cross Ref
Indexed at, Google Scholar, Cross Ref
Indexed at, Google Scholar, Cross Ref
Indexed at, Google Scholar, Cross Ref
Indexed at, Google Scholar, Cross Ref
Indexed at, Google Scholar, Cross Ref
Indexed at, Google Scholar, Cross Ref
Indexed at, Google Scholar, Cross Ref
Author Info
Bhavana Garapati1*, Sankari Malaiappan1, S Rajeshkumar2 and Karthikeyan Murthykumar1
1Department of Periodontics, Saveetha Dental College and Hospitals, Saveetha Institute of Medical and Technical sciences (SIMATS), Saveetha University, Chennai, India2Department of Pharmacology, Saveetha Dental College and Hospitals, Saveetha Institute of Medical and Technical sciences(SIMATS), Saveetha University, Chennai, India
Received: 10-Aug-2022, Manuscript No. jrmds-22-54117; , Pre QC No. jrmds-22-54117(PQ); Editor assigned: 12-Aug-2022, Pre QC No. jrmds-22-54117(PQ); Reviewed: 29-Aug-2022, QC No. jrmds-22-54117(Q); Revised: 01-Sep-2022, Manuscript No. jrmds-22-54117(R); Published: 08-Sep-2022