Research - (2022) Volume 10, Issue 7
Is Superantigen a Promising Immunotherapy Treatment Against Cancer?
S Al Zahrani1 and S EL Hadad1,2*
*Correspondence: S EL Hadad, Research Center of Genetic Engineering and Bioinformatics, VACSERA, Cairo, Egypt, Email:
Abstract
Superantigens are toxins produced in hosts by some pathogenic microbes as a mechanism to sustain their pathogenicity. Superantigens are highly resistant to degradation by proteases enzyme and heat denaturation and cause many dangerous diseases that in severe cases lead to death. Superantigens are atypical antigens that stimulate a profound proliferation of polyclonal T cells at low concentrations. All superantigens have a standard architecture even though they differ in amino acid sequences and are divided into two groups: endogenous superantigens and exogenous superantigens. The major histocompatibility complex molecules on antigen-presenting cells and T cell receptors on T cells are the major players in identifying foreign antigens. Superantigens can bind nonspecifically to both the major histocompatibility complex class II molecules and T cell receptors and form a trimolecular complex. Superantigens do not follow conventional antigens processing and presentation; they bind as intact macromolecules outside the antigen-binding groove of the major histocompatibility complex class II and to Vβ of T cells receptors. Thus, triggering an excessive release of proinflammatory cytokines. Radiotherapy and chemotherapy often develop radio/drug resistance. Hence, cancer immunotherapy has been mainly considered as it instigates the patient’s immune system to fight cancer. Superantigens are one of the most potent T cell mitogens as 0.1 pg/ml is adequate to excite T lymphocytes. Accordingly, extensive in-vivo/in-vitro investigations have been conducted on the potential role of superantigens in eradicating tumors. The safety and effectiveness of superantigens as a cancer treatment have been verified in many clinical trials. Nevertheless, the vast inflammation after the potent T cell activation is known to promote diseases, including cancer. This paper reviews the Potentiality of superantigen as an immunotherapy treatment against cancer.
Keywords
Superantigens, Enterotoxins, T cell, MHC, Immunotherapy, Cancer, Proinflammatory cytokines
Introduction
Superantigens (SAgs) are toxins produced in hosts by pathogenic microbes such as bacteria, viruses, and mycoplasma. SAgs are a leading cause of many dangerous diseases such as toxic shock syndrome (TSS), food poisoning, and scarlet fever [1]. SAgs are highly resistant to degradation by proteases enzyme and heat denaturation. They vary in size from 22 to29 kDa and are absorbed by the epithelium as intact proteins [2]. SAgs are atypical antigens that stimulate a profound proliferation of human and other mammalian T cells; this stimulation occurs at a very low superantigens concentration compared to conventional antigens [3,4]. In One theory, microbes produce SAgs to maintain their pathogenicity and colonization in the host as SAgs convey the immune response away from the microbe’s colonies by eliciting cytokine responses that disrupt the activity of the effector cells (cells responsible for the clearance of pathogens) as reported by Tuffs et al. [5].
SAgs differ in the amino acid sequences, but all have a typical architecture. SAgs consist of two domains: aminoterminal domain and carboxy-terminal domain. The two parts are spanned with a long solvent-accessible α -helix in the center and have a zinc-binding site. The aminoterminal domain consists of a β -barrel, and α -helix, exposed hydrophobic residues, and a disulfide bridge. The Carboxy-terminal domain consists of four β -sheets flanked by and α-helix [6]. The Zinc-binding site is found in most SAgs, and it has a direct effect on the recognition of superantigens by MHC class II molecules [7].
Superantigens bind MHC class II molecules and T cell receptors
One of the properties of Superantigens is their unique ability to bind non-specifically to both major histocompatibility complex (MHC) class II molecules on antigen-presenting cells (APC) and T cell receptors (TCR) on T cells. The binding of SAg forms a trimolecular complex that activates more resting T cells (1 in 5 T cells) than the conventional antigens, 1 in 1000 T cells [8].
One of the key players in identifying foreign antigens is MHC, also called the human leukocyte antigen (HLA). MHC molecules play an essential role in both cell-mediated and humoral immunity. MHC is a large region of the vertebrate DNA and comprises hundreds of alleles. In humans, the MHC region is located on chromosome 6, and it encloses 224 genes; these genes express proteins involved in antigen processing and presentation. MHC genes that deliver specific antigens to T lymphocytes are classified into MHC classes I and II. MHC genes are essential for the adaptive immune system as they are expressed on the cell surface and are called MHC molecules. MHC molecule present antigens on the cell surface to help specify the antigens by the adaptive immune system and supports the elimination of abnormal/infected cells [9,10].
The roles of both MHC class I and class II molecules are to present peptides at the cell surface to CD8+ and CD4+ T cells, respectively. Nevertheless, the mechanisms of antigens processing and presentation by both MHC class I and II molecules differ and are well-determined [11]. Type I MHC is found on all nucleated cells, and it presents peptides from all intracellularly expressed proteins. Abnormality in one of these proteins activates CD 8 lymphocytes that eliminate the cell through apoptosis. Whereas MHC Class II is located on some types of immune cells known as antigen-presenting cells or APC such as macrophages, monocytes, and dendritic cells. The role of APC is engulfing encountered pathogen and presenting part of it to the appropriate T cell. T cell then evokes different responses and alerts the immune system of the threat. Thus, MHC Class II present peptides derived from foreign proteins only [12].
Pathogens from external sources are engulfed and processed intracellularly in the cytoplasmic phagolysosome; a single peptide (antigen) then is loaded on MHC class II molecules and transported to the cell surface [13]. TCR on T cell recognizes and binds to the antigen epitope on the HLA complex of APC. The co-stimulatory signals B7-2/CD28 are required to maintain a reasonable and adequate immune response to minimize unnecessary effects [14].
Another key player in the identification of antigens is T cells. T cells, types of white blood cells (leukocytes), have a crucial role in the adaptive immune response. CD4+ T cells are one of the most important immune cells for their production of different types of cytokines and chemokine’s that help in assisting B cells to make antibodies, stimulating macrophages microbicidal activity, and attracting neutrophils to the site of infection [15]. CD4+ T cells are divided into T regulatory cells (Treg) and T helper (Th) cells. As their names imply, Th cells help the adaptive immune system activate other effector cells such as B cells, macrophages, and CD8+ cytotoxic T cells. While Treg cells maintain self-tolerance by blocking Th cells' harmful activities that may cause autoimmune diseases [16]. CD4+ T cells are the primary targets of superantigens as the activation of CD4+ T cells results in the production of T helper type 1 (Th1) cytokine that suppresses the production of antibodies and increases the invading microbe [7].
T cells recognize viruses /bacterial invading and cancerous cells through their cell surface receptors that are called T cell receptors (TCRs). Most cell-surface receptors recognize a single ligand, whereas each TCR recognizes distinct ligands with different affinities [17]. TCR has either α/β or γ/δ heterodimers (90% of peripheral blood T cells have α/β peptide chain) that recognize and bind antigens presented by MHC molecules on APC. Another complex involved in the activation of TCR is the cluster of differentiation 3 (CD3) complexes that consists of four distinct subunits (epsilon (ε), gamma (γ), delta (δ), and zeta (ζ)). These peptides assemble and form three dimers (εγ, εδ, ζζ). All CD3 subunits possess immunoreceptor tyrosine-based activation motifs (ITAMs) in their cytoplasmic domains [18].
The conventional recognition of foreign antigens differs from the recognition of superantigens. In the conventional recognition of antigen, the α and β variable regions of the TCR must interact with the antigen that is processed intracellularly and presented on the major groove of the MHC molecule on the surface of APC (Figure 1). After the APC produces a co-stimulatory signal, biochemical changes start in the cytoplasmic region of the CD3 complex and stimulate an immune synapse (IS) between T cells and APCs. These synapses activate a small fraction of T cells, <0.01% [19]. However, Superantigens differ from conventional antigens in various aspects. Superantigens do not process intracellularly; instead, they bind as an intact macromolecule to APC and T cells. SAgs bind from one end to conserved residues on MHC class II molecules outside the antigen-binding groove of APC and from the other end to the V β regions on TCR forming a complex of APC-SAg-T cell (Figure 1). Thus, activating a high frequency of responding T cells (up to 20% of all T cells). MHC class II molecules have two different superantigen binding sites. One is a low-affinity binding site called the generic site found on the α-chain of the MHC class II molecule. The second is a high-affinity zinc-dependent site on the β-chain [7]. Superantigens are not processed intracellularly and do not fit in the MHC II binding groove; thus, bypassing the conventional antigen processing and presentation [6,20].
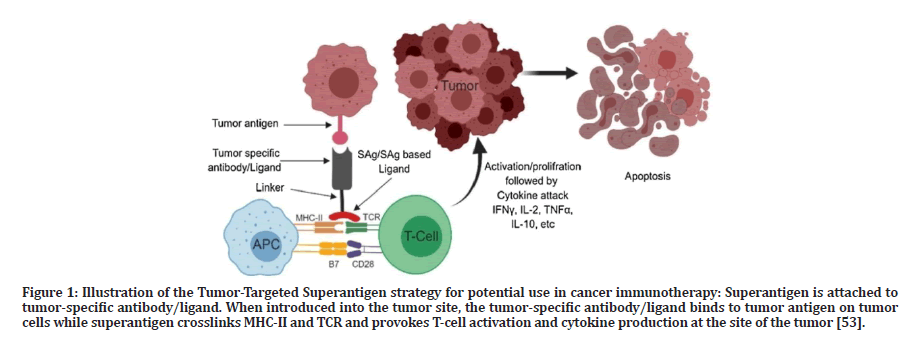
Figure 1: Illustration of the Tumor-Targeted Superantigen strategy for potential use in cancer immunotherapy: Superantigen is attached to tumor-specific antibody/ligand. When introduced into the tumor site, the tumor-specific antibody/ligand binds to tumor antigen on tumor cells while superantigen crosslinks MHC-II and TCR and provokes T-cell activation and cytokine production at the site of the tumor [53].
The interaction between CD28 and its B7-2 co-ligand is usually weak; yet, It is found that superantigens induce production of cytokine storm not only by the interaction between MHC-II molecule and T-cell receptor but also by enhancing the engagement of the B7-2/CD28 coreceptor, which turned out to be critical superantigen receptor [21]. The design of short peptides that mimic B7-2/ CD28 dimer block the binding of superantigen to these receptors and protects from the lethal shock caused by the over-stimulation of immune cells [22].
Types of superantigens
There are two types of superantigens: the first type is endogenous superantigens. Endogenous superantigens are the product of retroviruses that invade hosts and integrate into the genome. Once combined, they are expressed as a part of the host genome. One example of endogenous superantigens is the mouse mammary tumor virus (MMTV), a B-type retrovirus transmitted through the milk to offspring and joined into the germline causing murine mammary carcinoma. The MMTV DNA encodes endogenous SAg called the minor-lymphocytesstimulating (MLS). It is the first superantigen described by Festenstein in 1973 as it stimulates a significant fraction of the T cells [23]. Another example of endogenous superantigens is the Epstein-Barr virus (EBV). This human herpesvirus encodes over 100 genes, is transmitted via saliva, and infects nasopharyngeal epithelial cells and B lymphocytes. The infection of EBV usually occurs in early childhood with no consequences until adolescence, where the EBV develops to infectious mononucleosis, IM [24].
The second type of SAg is exogenous superantigens. Exogenous superantigens are produced by microbes such as Staphylococcus aureus and Streptococcus pyogenes [24]. Bacterial superantigens are the most potent activators of T cells. In vitro investigations indicates that picograms concentrations of bacterial superantigens can stimulate huge proliferation of peripheral T cells and massive cytokine production [5].
Staphylococcus enterotoxin highly stimulates the immune system and has many immunological activities [25]. The bacterium, S. aureus, gram-positive bacteria, are one of the normal and harmless microbiotas found in the human nasopharynx. It considers pathogenic once the skin, the primary barrier that isolates it from the bloodstream, is penetrated. Staphylococcal superantigens cause serious diseases such as food poisoning, toxic shock syndrome, staphylococcal scalded skin syndrome. Staphylococcal aureus can produce up to 24 types of superantigens divided into three groups: enterotoxins, exfoliative toxins, and TSST- 1. Enterotoxins are made under certain conditions in foods and culture media and involve seven types (SEA, SEB, SEC 1, SEC2, SEC3, SED, SEE); all enterotoxins have a high ability to stimulate T cells [26].
Studies from various mammalian species have shown that bacterial superantigens such as staphylococcus enterotoxin provoke nonspecific activation of lymphocytes. Because bacterial superantigens excite many T cells, they are called T-cell mitogens. After the MHC II /superantigen complex bind to T cells, a massive release of a wide range of cytokines occurred, such as interferon-gamma, tumor necrosis factor (TNF), interleukin 2 (IL-2), interleukin 4 (IL-4), and interleukin 6 [20]. Therefore, the stimulation of T cells can be inhibited by the addition of antibodies to MHC class II molecules [27]. All Enterotoxins bind with different affinities to MHC class II and TCR [28].
Superantigens and proinflammatory cytokines
Cytokines are proteins usually produced by immune cells and act as ligands that bind to specific receptors on effector cells to stimulate the proliferation, differentiation, or drive inflammatory or antiinflammatory responses [29]. Superantigens trigger excessive cytokines, especially proinflammatory cytokines such as IFNγ, TNF-α, IL-1β, IL-6 [30]. The massive release of proinflammatory cytokines caused by SARS-CoV-2 (Coronavirus) SAgs is believed to be the reason for the systemic shock, acute respiratory syndrome, and multiorgan failure occurrence in patients with severe COVID-19 [31].
IFNγ is the main cytokine produced in response to SAg/T cell interaction. IFNγ is one of the interferon proteins family that aids host resistance to microbial infection and plays a critical role in immune/inflammatory responses and tumor immunosurveillance. It is produced by NK cells and specific CD4+ and CD8+ subsets of T cells following immune and inflammatory stimuli. The production of IFNγ regulates cell-mediated immune responses; Th1 cells produce IFNγ to enhance macrophages and cytotoxic CD8+ T cells. IFNγ prevents the formation of many primary and transplanted tumors in mice. It stimulates host responses to tumors by affecting tumor survival, blocking tumor angiostasis, or enhancing the innate and adaptive immune responses against tumors [32]. IFNγ has effects on not only immune cells but somatic cells and tumor cells. It prevents tumor development through its ability to slow tumor cell proliferation, up-regulate the expression of MHC class I and components of the antigen-processing mechanism, induce signals in T cells for increasing effectiveness, and induce apoptosis. The binding of IFNγ to its receptor consists of two parts, IFNgR1 and R2, on the cell surface. This causes the receptor to dimerize and activate downstream signaling, Such as Janus kinase (JAK)–signal transducer and transcription 1 (STAT1) pathway, by trans phosphorylation. Downstream signaling then regulates transcription and all cellular changes associated with IFNγ [33]. A high level of IFNγ helps S. aureus persist and proliferate during systemic infection [34].
Tumour necrosis factor-alpha (TNFα) is another cytokine made by inflammatory cells, mainly macrophages, and plays a significant role in the immune system and tumor surveillance. TNFα has two binding sites, one on the TNFR1 receptor, which is expressed in most tissues as a death receptor, and the other on TNFR2 expressed in immune cells. The binding of TNF α to its receptor activates three central signaling cascades: the nuclear factor kappa B (NF- κB) pathway, the mitogenactivated protein kinase (MAPK) pathway, and the stimulation of death signaling. TNF-α induces cancer cell death by activating antigen-presenting cells, enhancing proliferation of effector T cells, and initiating massive hemorrhagic necrosis of transplanted tumors. TNF α binds to tumor cells, endothelial cells, blood vessels, and normal cells and provokes damage to many cells types. Likewise, TNF-α stimulates Th9 cell differentiation which induces further potent inhibition of tumor growth in mouse models [35,36].
IL-6 is produced by innate immune cells such as macrophages and monocyte following infections or tissue damages. IL-6 plays many critical roles, such as stimulating B cells to produce immunoglobulin (Ig), inducing acute phase proteins production, prompting hematopoietic stem cell differentiation, and regulating specific differentiation of naive CD4+ T cells. IL-6 enhances angiogenesis and increases vascular permeability by inducing the production of vascular endothelial growth factor VEGF [37].
IL-1β is produced by various cell types, including immune cells, fibroblasts, and cancer cells. The production of IL- 1β involves two processes, called priming and cleavage. High levels of IL1B mRNA in breast cancer patients are associated with a better prognosis than patients with low levels. Also, cervical cancer women with common IL1B expression exhibited an increase in the risk of progression of pre-neoplastic lesion [38].
Superantigen is a potential immunotherapy treatment against cancer
Immunotherapy is a cancer treatment used to stimulate antitumor immune cells such as CD8+ cytotoxic T cells, Th1 cells, Th2 cells, natural killer (NK) cells. These cells induce the killing of cancer cells through apoptosis; or the release of antitumor molecules that cause cancer cell death. Radiotherapy/chemotherapy treatment often devolves radio/drug resistance. Moreover, surgical treatment is limited to some cases, making immunotherapy an excellent alternative treatment [39]. SAgs are one of the most potent T cell mitogens as 0.1 pg/ml is adequate to excite T lymphocytes. Accordingly, SAgs can be used in cancer treatment as immunemodifier agents. Superantigens have exhibited a robust antitumor outcome in animal testing and clinical trials as they are potent immunomodulators and efficient cytokine inducers.
Chemotherapeutic drugs, surgery, and radiation treat cancers, although not all are susceptible to these methods. Therefore, there has been a demand for newer techniques to treat cancers [7]. Various superantigens possess immunomodulatory activities that either reduce or kill cancer cells through enhancing the immune system’s attack toward tumors. The antitumor activity of some bacterial superantigens have been tested on many cancer cell lines such as human hepatoma cell carcinoma (BEL-7402, SMMC 7721, Hepa-2), human colon adenocarcinoma (HT-29), human stomach adenocarcinoma (BGC-823), human cervix tumor cell line (Hela), oral squamous carcinoma (KB,) human lung adenocarcinoma (GLC-82, and A549), and human breast adenocarcinoma (MCF-7 and ZR-75-1), and others. In the in vitro investigations, the peripheral blood mononuclear cells (PBMCs), the source of T cells, were isolated from healthy blood donors then were stimulated by superantigen such as SEB, SEC1, SEA-H61D. Stimulated PBMCs are then co-cultured with the cancer cell line of investigation, and the cytotoxicity of the stimulated PBMCs is measured using an MMT assay. It is longestablished that Superantigens speed up the proliferation of PBMCs and induce the expressions of cytokines. As the percentage of stimulated PBMC expanded, the inhibition of cancer cells increased [40,41]. SEC1 stimulates PBMCs proliferation in a dose-dependent manner and excites the release of interleukin-2, interferon-γ, and tumor necrosis factor-α. The stimulated PBMCs exhibits high cytotoxicity toward the human bladder cancer cells in vitro. Also, SEC1 decreases tumor formation rate and increases tumor-bearing mice survival in vivo [42]. In vivo studies of the oral administration of SEC2 indicated that SEC2 crosses the intestinal epithelium as an intact form and maintains its antitumor activity by stimulating T cells [43]. The anti-tumor effect of staphylococcal enterotoxin C (SEC) combined with other treatments has been investigated on patients with gliomas. The gliomas had been surgically removed, and patients were treated with a systemic application of SEC in combination with chemotherapy and radiotherapy. The clinical data indicates that the effective rate of surgery, radiotherapy, and chemotherapy is only 32.0%. In comparison, a combined treatment that involves chemotherapy plus SEC increases the effective rate to 63.6% [44]. SAM-3, a mutated version of staphylococcal enterotoxin c subtype 2 (SEC2), exhibits improved superantigen activity with lower toxicity than native SEC2. SAM-3 inhibits the growth of H22 and Hepa1-6 tumor cells in vitro and colon 26 solid tumors in vivo [45].
Staphylococcal enterotoxin B (SEB) elicits vigorous immune activities that inhibit tumor growth in vivo and in vitro. The injection of appropriate doses of SEB in animal models eliminates various types of tumor cells [10]. Treatment of SEB provokes many immune activities in SqC-bearing mice, including induction of Th9 cells level, which plays a role in inducing apoptosis in SqCbearing mice. TGFαL3-SEB chimeric protein induces cell death in HT-29 colon cancer cells [34]. The antitumor activity of TGFαL3-SEB was also investigated in mice bearing breast cancer. The intratumoral administration of TGFαL3-SEB induces necrosis and suppresses the proliferation of the growth of breast tumors through stimulating the release of high interferon γ (IFN-γ) and tumor necrosis factor α (TNF-α) [46].
Nevertheless, a recent systematic review indicates that Staphylococcal enterotoxin A was the most used toxin in studies involving the usage of superantigens as cancer treatment and that due to the reduced side effects of this toxin [47]. SEA is one of the staphylococcus toxins that cause food poisoning, and it accounts for about 80% of food poisoning outbreaks cases in the USA. SEA, unlike other enterotoxins, has two binding sites for major histocompatibility complex (MHC) class II molecules. Further, SEA is the most effective among all studied toxins as it provokes polyclonal T cell proliferation. Picomolar amounts of SEA are enough to stimulate cytotoxic activity against many cancer cell lines [23]. SEA can be used as a novel protein drug for cancer immunotherapy since it has a strong affinity for human MHC class II and is considered a potent activator of T lymphocytes. When SEA is co-cultured with human peripheral blood mononuclear cells (PBMCs), it inhibits the proliferation of human lung carcinoma A549 cells, induces apoptosis, stimulates and increases the release of a wide range of proinflammatory cytokines [41].
Superantigens eliminate cancer cells through many critical biological processes. The most important is apoptosis; superantigens activate T cells and induce massive production of essential cytokines, including tumor necrosis factors (TNFs), interferons (INFs), and interleukins (Ils). These cytokines assist the destruction of the tumor’s vascular endothelial cells, help in leukocyte migration to cancer tissue, increase T lymphocytes differentiation, and accelerate the tumor elimination. Superantigens stimulate apoptosis by inducing FASL molecules on the surface of the PBMCs50 [48]. Superantigens also help in eradicating cancer cells through cancer clearance mechanisms. The interleukins produced by T cells activate the lymphokine-activated killer (LAK) cells that can eliminate resisted tumor cells. Moreover, superantigens prevent tumor cell metastasis by activating NK cells that subvert the cancer cell membranes and provoke apoptosis [49].
Superantigens can be used as vaccines or adjuvants to augment T cell activation. Injection of superantigens in mice before tumor transplantation triggers the killing of a large fraction of the tumor and increases the survival of mice [50]. Superantigens can also increase chimeric antigen receptor (CAR) T cell activity. Chimeric antigen receptor (CAR) T cell is a therapy in which patient’ T cells are modified to produce chimeric antigen receptors (CARs) on their surface. CAR T-cell therapy is a very effective treatment for some leukemias and lymphomas but less applicable for solid tumors. However, the combination of CAR T cell transfer with the staphylococcal enterotoxin-B (SEB) considerably enhances the function and proliferation of CAR T cells. It increases the inhibition of solid tumor growth in mice [51].
Few SAgs have been tested as cancer treatments in clinical trials. The Fab-SEA fusion protein (PNU-214565) is one of the modified SAgs tested in a clinical trial. SEA was fused with the Fab fragment of the monoclonal antibody C242 that recognizes human colorectal (CRC) and pancreatic carcinomas (PC). Treatment with PNU- 214565, a SAg-based tumor-targeted therapy, is found to be effective and safe with a maximum dose of up to 1.5 ng/kg [52]. Also, the Intertumoral injection of SEC in hepatocellular carcinoma (HCC) patients was efficient. Naptumomab estafenatox (antibody-superantigen fusion protein) is a drug under development, and it will be used to treat some types of cancer, such as renal cell carcinoma. Clinical testing showed that treatment with Naptumomab estafenatox induced T-cell mediated killing of tumor cells. Free superantigens cause severe side effects as they potently activate nonspecific human T cells. Accordingly, tumor-targeted superantigens (TTS) have been established. This strategy attracts the immune response toward tumors by linking superantigens to cancer-specific antibodies/ ligands. Thus, it brings T cells in great proximity to tumor cells [5]. Tumortargeted superantigens (TTS) eradicate more than 80% of tumor cells in mice models of melanoma. Hence, the powerful properties of superantigens can be harnessed to generate long-term immune responses to the cancer.
Superantigen may not be a potential immunotherapy treatment against cancer
The immunomodulatory activity of bacterial superantigens has been well-known for their effectiveness on many cancer cell lines. Nonetheless, superantigens are more recognized for their role in diseases. The considerable inflammation after the potent T cell activation promotes conditions, including cancer. Inflammation aids tumors progression as it is linked to cellular transformation, promotion, invasion, angiogenesis, and metastasis of tumors [54]. IFNγ, one of the proinflammatory cytokines produce via T cell activation, has a crucial role in eradicating a variety of tumors. The studies have shown that IFNγ increases growth, invasiveness, angiogenesis, and metastasis of the tumors. IFNγ enhances the growth of Papilloma by promoting the inflammatory reaction of Th17. IFNγ was also a critical factor in the development of colorectal carcinomas in SOCS1-deficient mice. Also, the activation of monocyte/macrophage by IFNγ triggers DNA damage in hepatocytes through the diethylnitrosamine-induced hepatocarcinogenesis [53].
The local expression of TNF-α has a therapeutic effect on cancer; Nonetheless, once TNF-α enters the circulation, it causes many diseases, including cancer. TNF-α is produced not only by macrophages but also by a wide variety of tumor cells. TNF-α produced by tumor cells could bind to the same cell (autocrine) and act as a growth factor or stimulate the expression of other growth factors that mediate tumors proliferation [54]. TNF-α expression correlates with tumor progression. The level of TNF-α is associated with the lymph node metastasis and the breast cancer stage . TNF-α also enhances the progression of colon cancer stem cells [55].
Reactive oxygen/nitrogen species (ROS/RNS) are produced from inflammatory and epithelial cells during chronic inflammation. (ROS/RNS) damage nucleic acids, proteins, and lipids and trigger tissues/organs damage. Approximately 25% of cancers develop from infection and inflammation. The inflammatory microenvironment affects the down regulation of tumor suppressor genes and microRNAs. The exposure to ROS/ RNS and interleukin 6 (IL-6) produced from inflammatory cells silence the transcription of DNA methyltransferase 1 (DNMT1) protein, by hyper methylation which plays a role in the carcinogenesis [30].
References
- Reiser RF, Jacobson JA, Kasworm EM, et al. Staphylococcal enterotoxin antibodies in pediatric patients from Utah. J Infect Dis 1988; 158:1105–1108.
- Papageorgiou AC, Acharya KR. Microbial superantigens: From structure to function. Trends Microbiol 2000; 8:369–375.
- Marrack P, Kappler J. The staphylococcal enterotoxins and their relatives. Science 1990; 248:705-711.
- Chen JY. Superantigens, superantigen-like proteins and superantigen derivatives for cancer treatment. Eur Rev Med Pharmacol Sci 2021; 25.
- Tuffs SW, Haeryfar SM, McCormick JK. Manipulation of innate and adaptive immunity by staphylococcal superantigens. Pathogens 2018; 7:53.
- Agrawal R, Kamboj D V. A review on superantigens. 2012.
- Baker MD, Acharya KR. Superantigens. Superantigen Protoc 2003; 1–31.
- Baker MD, Acharya KR. Superantigens: Structure-function relationships. Int J Med Microbiol 2004; 293:529–537.
- Wen R, Cole GA, Surman S, et al. Major histocompatibility complex class II-associated peptides control the presentation of bacterial superantigens to T cells. J Exp Med 1996; 183.
- Sur G, Sporis D, Kudor-Szabadi L, et al. Super-antigens and human pathology: Always an interesting topic. J Bioequivalence Bioavailab 2013; 5.
- Neefjes J, Jongsma MLM, Paul P, et al. Towards a systems understanding of MHC class I and MHC class II antigen presentation. Nat Rev Immunol 2011; 11:823–836.
- Vyas JM, Van der Veen AG, Ploegh HL. The known unknowns of antigen processing and presentation. Nat Rev Immunol 2008; 8:607–618.
- Zumla A. Superantigens, t cells, and microbes. Clin Infect Dis 1992; 15.
- Vaishnani J. Superantigen. Indian J Dermatology, Venereol Leprol 2009; 75:540.
- Zhu J, Paul WE. CD4 T cells: Fates, functions, and faults. J Am Soc Hematol 2008; 112:1557–1569.
- Corthay A. How do regulatory T cells work? Scand J Immunol 2009; 70:326–336.
- Janeway CA, Travers P, Walport et al. The components of the immune system. In: Immunobiology: The Immune System in Health and Disease 5th Ed. Garland Science 2001.
- Goyette J, Nieves DJ, Ma Y, et al. How does T cell receptor clustering impact on signal transduction? J Cell Sci 2019; 132:226423.
- Gaud G, Lesourne R, Love PE. Regulatory mechanisms in T cell receptor signalling. Nat Rev Immunol 2018; 18:485–497.
- Jett M, Das R, Neill R. The staphylococcal enterotoxins. In: Molecular medical microbiology. Elsevier 2002; 1089–116.
- Levy R, Rotfogel Z, Hillman D, et al. Superantigens hyperinduce inflammatory cytokines by enhancing the B7-2/CD28 costimulatory receptor interaction. Proc Natl Acad Sci 2016; 113:e6437.
- Kaempfer R. Bacterial superantigen toxins, CD28, and drug development. Toxins 2018; 10:459.
- Lando PA, Hedlund G, Dohlsten M, et al. Bacterial superantigens as anti-tumour agents: Induction of tumour cytotoxicity in human lymphocytes by staphylococcal enterotoxin A. Cancer Immunol Immunother 1991; 33:231–237.
- Rajagopalan G, Singh M, Sen MM, et al. Endogenous superantigens shape response to exogenous superantigens. Clin Vaccine Immunol 2005; 12:1119–1122.
- Tapeh BEG, Hashemzadeh MS, Hoseini AM. The role of bacterial superantigens in the immune response: From biolo-gy to cancer treatment. Curr Cancer Ther Rev 2020; 16:1.
- Vasconcelos NG, de Souza M de LR. Staphylococcal enterotoxins: Molecular aspects and detection methods. J Public Heal Epidemiol 2010; 2:29–42.
- Misfeldt ML. Microbial" superantigens". Infect Immun 1990; 58:2409.
- Hudson KR, Tiedemann RE, Urban RG, et al. Staphylococcal enterotoxin A has two cooperative binding sites on major histocompatibility complex class II. J Exp Med 1995; 182:711–720.
- Berraondo P, Sanmamed MF, Ochoa MC, et al. Cytokines in clinical cancer immunotherapy. Br J Cancer 2019; 120:6–15.
- Murata M. Inflammation and cancer. Environ Health Prev Med 2018; 23:1–8.
- Scaglioni V, Soriano ER. Are superantigens the cause of cytokine storm and viral sepsis in severe COVID-19? Observations and hypothesis. Scand J Immunol 2020; 92.
- Ikeda H, Old LJ, Schreiber RD. The roles of IFNγ in protection against tumor development and cancer immunoediting. Cytokine Growth Factor Rev 2002; 13:95–109.
- Ni L, Lu J. Interferon gamma in cancer immunotherapy. Cancer Med 2018; 7:4509–4516.
- Tuffs SW, Goncheva MI, Xu SX, et al. Superantigens promote Staphylococcus aureus bloodstream infection by eliciting pathogenic interferon-gamma (IFNγ) production that subverts macrophage function. Bio Rxiv 2021.
- Shen J, Xiao Z, Zhao Q, et al. Anti‐cancer therapy with TNF α and IFN γ: A comprehensive review. Cell Prolif 2018; 51:e12441.
- Jiang Y, Chen J, Bi E, et al. TNF-α enhances Th9 cell differentiation and antitumor immunity via TNFR2-dependent pathways. J Immunother Cancer 2019; 7:1–12.
- Tanaka T, Narazaki M, Kishimoto T. Interleukin (IL-6) immunotherapy. Cold Spring Harb Perspect Biol 2018; 10:a028456.
- Rébé C, Ghiringhelli F. Interleukin-1β and cancer. Cancers 2020; 12:1791.
- Miao BP, Zhang RS, Sun HJ, et al. Inhibition of squamous cancer growth in a mouse model by Staphylococcal enterotoxin B-triggered Th9 cell expansion. Cell Mol Immunol 2017; 14:371–379.
- Wang HR, Jiang H, Hao HJ, et al. In vivo and in vitro antitumor effects of a staphylococcal enterotoxin a mutant (SEA-H61D). Cancer Invest 2010; 28:788–796.
- Liu T, Li L, Yin L, et al. Superantigen staphylococcal enterotoxin C1 inhibits the growth of bladder cancer. Biosci Biotechnol Biochem 2017; 81:1741–1746.
- Liu X, Zeng L, Zhao Z, et al. PBMC activation via the ERK and STAT signaling pathways enhances the anti-tumor activity of Staphylococcal enterotoxin A. Mol Cell Biochem 2017; 434:75–87.
- Zhao W, Li Y, Liu W, et al. Transcytosis, antitumor activity and toxicity of staphylococcal enterotoxin C2 as an oral administration protein drug. Toxins 2016; 8:185.
- Wang F, Huang Q, Zhou LY. Analysis of the treatment of gliomas with SEC therapy combined with radiochemotherapy. Age 2015; 23:22–72.
- Xu M, Wang X, Cai Y, et al. An engineered superantigen SEC2 exhibits promising antitumor activity and low toxicity. Cancer Immunol Immunother 2011; 60:705–713.
- Yousefi F, Siadat SD, Saraji AA, et al. Tagging staphylococcal enterotoxin B (SEB) with TGFaL3 for breast cancer therapy. Tumor Biol 2016; 37:5305–5316.
- Shivaee A, Sedighi M, Fooladi AAI. Staphylococcal enterotoxins as good candidates for cancer immunotherapy: A systematic review. Ann Di Ig Med Prev E Di Comunita 2020; 32:648–663.
- Hedlund G, Dohlsten M, Lando PA, et al. Staphylococcal enterotoxins direct and trigger CTL killing of autologous HLA-DR+ mononuclear leukocytes and freshly prepared leukemia cells. Cell Immunol 1990; 129:426–434.
- Tian XL, Yan Z, Chen J, et al. Clinical application of highly agglutinative staphylococcin in cancer treatment updates of the literature. Eur Rev Med Pharmacol Sci 2016; 20:2718–2725.
- Kominsky SL, Torres BA, Hobeika AC, et al. Superantigen enhanced protection against a weak tumor‐specific melanoma antigen: Implications for prophylactic vaccination against cancer. Int J Cancer 2001; 94:834–841.
- von Scheidt B, Wang M, Oliver AJ, et al. Enterotoxins can support CAR T cells against solid tumors. Proc Natl Acad Sci 2019; 116:25229.
- Giantonio BJ, Alpaugh RK, Schultz J, et al. Superantigen-based immunotherapy: A phase I trial of PNU-214565, a monoclonal antibody-staphylococcal enterotoxin A recombinant fusion protein, in advanced pancreatic and colorectal cancer. J Clin Oncol 1997; 15:1994–2007.
- Bashraheel SS, Domling A, Goda SK. Update on targeted cancer therapies, single or in combination, and their fine tuning for precision medicine. Biomed Pharmacother 2020; 125:110009.
- Aggarwal BB, Shishodia S, Sandur SK, et al. Inflammation and cancer: How hot is the link? Biochem Pharmacol 2006; 72:1605–1621.
- Wei X, Li X, Kong F, Ma L, Sui Y, Chen D, et al. TNF-α activates Wnt signaling pathway to promote the invasion of human colon cancer stem cells. Chinese J Cell Mol Immunol 2018; 34:982–988.
Indexed at, Google Scholar, Cross Ref
Indexed at, Google Scholar, Cross Ref
Indexed at, Google Scholar, Cross Ref
Indexed at, Google Scholar, Cross Ref
Indexed at, Google Scholar, Cross Ref
Indexed at, Google Scholar, Cross Ref
Indexed at, Google Scholar, Cross Ref
Indexed at, Google Scholar, Cross Ref
Indexed at, Google Scholar, Cross Ref
Indexed at, Google Scholar, Cross Ref
Indexed at, Google Scholar, Cross Ref
Indexed at, Google Scholar, Cross Ref
Indexed at, Google Scholar, Cross Ref
Indexed at, Google Scholar, Cross Ref
Indexed at, Google Scholar, Cross Ref
Indexed at, Google Scholar, Cross Ref
Indexed at, Google Scholar, Cross Ref
Indexed at, Google Scholar, Cross Ref
Indexed at, Google Scholar, Cross Ref
Indexed at, Google Scholar, Cross Ref
Indexed at, Google Scholar, Cross Ref
Indexed at, Google Scholar, Cross Ref
Indexed at, Google Scholar, Cross Ref
Indexed at, Google Scholar, Cross Ref
Indexed at, Google Scholar, Cross Ref
Indexed at, Google Scholar, Cross Ref
Indexed at, Google Scholar, Cross Ref
Indexed at, Google Scholar, Cross Ref
Indexed at, Google Scholar, Cross Ref
Indexed at, Google Scholar, Cross Ref
Indexed at, Google Scholar, Cross Ref
Indexed at, Google Scholar, Cross Ref
Indexed at, Google Scholar, Cross Ref
Indexed at, Google Scholar, Cross Ref
Indexed at, Google Scholar, Cross Ref
Indexed at, Google Scholar, Cross Ref
Indexed at, Google Scholar, Cross Ref
Indexed at, Google Scholar, Cross Ref
Indexed at, Google Scholar, Cross Ref
Indexed at, Google Scholar, Cross Ref
Indexed at, Google Scholar, Cross Ref
Indexed at, Google Scholar, Cross Ref
Indexed at, Google Scholar, Cross Ref
Indexed at, Google Scholar, Cross Ref
Indexed at, Google Scholar, Cross Ref
Author Info
S Al Zahrani1 and S EL Hadad1,2*
1Department of Biological Science, Faculty of Science, King Abdulaziz University, Jeddah, KSA2Research Center of Genetic Engineering and Bioinformatics, VACSERA, Cairo, Egypt
Received: 14-Jun-2022, Manuscript No. JRMDS-22-66570; , Pre QC No. JRMDS-22-66570 (PQ); Editor assigned: 16-Jun-2022, Pre QC No. JRMDS-22-66570 (PQ); Reviewed: 01-Jul-2022, QC No. JRMDS-22-66570; Revised: 06-Jul-2022, Manuscript No. JRMDS-22-66570 (R); Published: 13-Jul-2022