Research - (2019) Volume 7, Issue 6
Influence of Coefficient of Friction on Stress Distribution in Implant Components and Surrounding Bone
Mohamed Tharwat Hamed1,2*, Hisham Abdullah Mously1, Abou Bakr Hosam Hashem3 and Ghada Hussein Naguib4,5
*Correspondence: Mohamed Tharwat Hamed, Faculty of Dentistry, Department of Oral & Maxillofacial Prosthodontics, Saudi Arabia, Email:
Abstract
Introduction: The proper screw preload delivery is affected by several factors, among which the coefficient of friction is responsible for affecting the delivery of proper amount of preload torque. It is believed that the delivered preload increases due to reduction in coefficient of friction.
Aims: The study aims to investigate maximum von Misses equivalent stresses generated within different parts of the assembly of ɸ 4.1 mm and 10 mm long Straumann implant.
Material and Methods: The implant is inserted in place of the mandible first molar because of tightening screw. An analysis considered the screw surfaces as frictional and concluded that the frictional moment and the axial force opposed the advancement of the screw during its tightening. These loads were utilized for the implementation of three-dimensional finite element linear stress-strain analysis to compute the maximum equivalent stress generated within each part of the implant assembly to compare with the yield stress material of that part. The material used for retaining screw and the coefficient of friction between the screw surfaces were the variables of the study, including the abutment and implant surfaces.
Results and Discussion: Results were obtained for two assemblies having type III gold and Ti-6Al-4V titanium alloy screws each with 14 values of the friction coefficient.
Conclusion: The study concluded that stresses at the maximum tightening moment applied on the screw invariably decrease due to an increase in the coefficient of friction for all assembly parts.
Keywords
Screw tightening, Components of dental implants, Stress, Dental restorations
Introduction
In a screw retained implant-supported prosthesis, the implant system components are connected by a titanium abutment screw and a gold prosthetic screw. The titanium abutment screw connects the abutment to the implant fixture. The gold screw connects the prosthesis to the abutment. Once the system is assembled by screw tightening, any load on the implant assembly system is dispersed in the system, as well as, transferred to the surrounding bone. Both the titanium and the gold prosthetic screws are threaded screws, which are tightened by delivering the manufacturer recommended preload.
Haack et al. [1] reported that preload tightening stresses in dental implant abutment screws after application of the manufacturer's recommended tightening torques were 57.5% and 56% of the yield strengths for gold and titanium, respectively. Peixoto et al. [2] utilized a three-dimensional model to evaluate the effect of implant abutments material from which the abutment is manufactured on the stress distribution in a single unit implant-supported fixed prosthesis. The results of the study indicated no difference between both materials. It also indicated that preloading contributed for 77.89% of the stress and that the screw was the most intensely affected part of the system.
There are several factors, which affects proper screw preload delivery. Coefficient of friction is one of the factors affecting delivery of proper amount of preload torque [3,4]. Bulaqi et al. [3] reported that reducing the coefficient of friction increases the preload delivered. Therefore, the purpose of this study was to explore the variance in coefficient of friction on stress distribution in the dental implant assembly system and the surrounding bone.
Materials and Methods
Screw tightening analysis
The turning moment M; applied on the top surface of the head of a screw for its advancement against an opposing force parallel to its axis, Fa, has been analyzed. The screw thread has a mean radius rmean with a helix angle α. The coefficient of friction between the threads of the screw and its nut is μ. Figure 1 has presented a frictional inclined surface analogy for the screw thread during its tightening. The thread is folded horizontally along the circumference of its mean circle to as represented by an inclined surface, making an angle α with the horizontal direction. The analogy is commonly used for the force analysis of a power screw working against an axial force Fa. The moment Ms has been applied on the screw to overcome friction acting on its threaded surface by creating a tangential force Ft, which tries to move the screw downwards the inclined surface. Therefore;
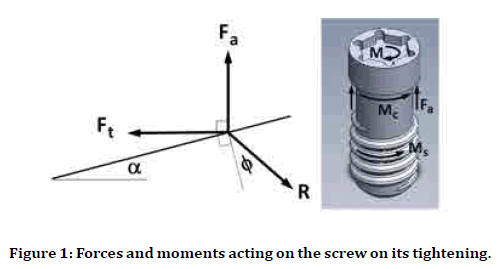
Figure 1. Forces and moments acting on the screw on its tightening.
Ms=Ft.rmean ….......................................………………….. (1)
The reaction of the nut thread surface on the screw thread surface was R, which deviates upwards from the direction normal to the inclined surface with the friction angle ɸ;
μ=tanф ...............................................................(2)
The three forces Ft, Fa, and R are in equilibrium
Ft=Fαtan(ф+α) ………………………………...(3)
Therefore
Ms=Fα.rmean tan(ф+α) ……………………….(4)
The applied moment with the torque wrench on the top of the screw head, Mc need to be more than Ms, where Mc is the frictional moment between the bottom surface of the screw head and its contact surface with the abutment. If the same coefficient of friction μ be invoked here, then
Mc=μFαRmean ………................…………………………… (5)
Where, Rmean is the mean radius of the contact area between the screw head and the abutment. Then
M=Ms+Mc=Fα[rmean tan(ф+α)+μ Rmean] …… ….(6)
After tightening the wrench moment, M is removed and hence Ms, Mc, and Ft do not exist. The screw was still subjected to the axial force Fa with its magnitude. To untighten the screw, the wrench was applied on the top of the screw head in the opposite direction of the tightening moment. The untightening moment is less than the tightening moment. The directions of the three moments M, Ms and Mc were reversed. In this case, the screw moves upward the inclined plane that the untightening moment Mu is given by
Mμ=Fα[rmean tan(ф+α)+μ Rmean] ……………………..(7)
Procedure
Solidworks Premium 2010 SP0.0 constructed a 3D geometrical model for a standard Ti-6Al-4V Straumann implant of ɸ4.1 mm and 12 mm long. The implants were inserted vertically in place of a missing mandible of an adult. The bone with the surrounding soft tissue extended for 14 mm along the local mesiodistal direction. The width of sagittal occlusal bone was approximately 11 mm. All other geometries of the cortical and cancellous bones and mucosa were considered accordingly in proportion. The cortical bone surrounded the core cancellous bone with a thickness of about 2 mm. The axis of the implant coincided with the midpoint of the occlusal table. After osseointegration, an integrated retaining screw fixed a standard Ti-6Al-4V abutment to the implant with a recommended tightening moment. An available failed implant was utilized for having as accurately as possible the dimensions of the implant including; the abutment and the screw. Figure 2 has presented an isometric view of the model with a corresponding sagittal cross section. The ANSYS Release 13.0 FEA software exported the geometry of the model to have a three-dimensional mesh generated. The material of each part defined the necessary constraints imposed and the loads applied on the implants. ANSYS generated the final mesh idealization with a smooth transition and a growth rate of 1.2. The mesh had 180398 nodes and 103928 iso-parametric elements. Mesh trials had been exercised to achieve convergence with that final mesh based on total elastic strain energy. Isotropic homogeneous linearly elastic materials were assumed. Table 1 has listed relevant mechanical properties of the materials used in the present work.
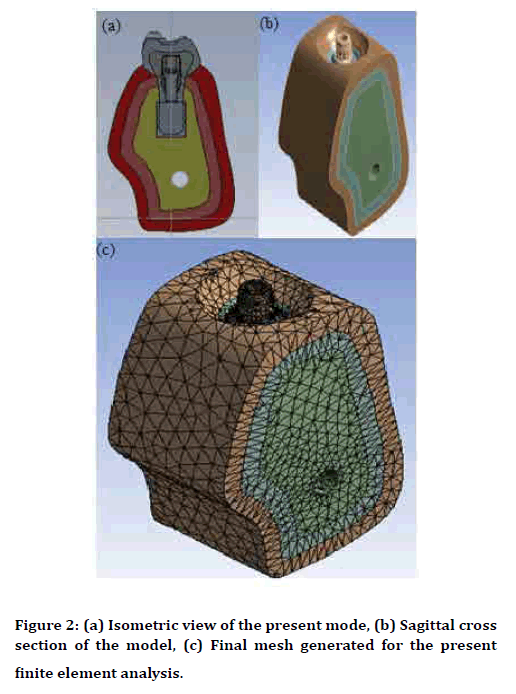
Figure 2. (a) Isometric view of the present mode, (b) Sagittal cross section of the model, (c) Final mesh generated for the present finite element analysis.
Material | Elasticity modulus (GPa) | Poisson’s ratio |
---|---|---|
Titanium alloy Ti-6Al-4V | 117 | 0.33 |
Type III Gold | 100 | 0.33 |
Cortical Bone | 14.7 | 0.3 |
Cancellous Bone | 0.49 | 0.3 |
Soft Tissue | 1.18 × 10-3 | 0.45 |
Table 1: Mechanical properties of the materials for the analyzed models.
The bottom surface of the cortical bone was fixed and mesiodistal displacements of both mesial and distal planes of the model were constrained. No separation was allowed at the contact surfaces of different parts of the assembly. The screw contact surfaces with the abutment and the implant were assumed to load the screw head for the simulation of its tightening. The screw has three loads, including
→ A tightening moment with its recommended value M on its top surface.
→ A frictional opposing moment Mc (equation 5) on its contact bottom surface.
→ Axial force Fa (equation 6) on its contact bottom surface opposing the advancement of the screw during its tightening.
Two screw materials of Ti-6Al-4V titanium alloy and type III gold were analyzed. The value of M was 30N.cm for the titanium screw and 20 N.cm for the gold screw. The present work considered 14 values of μ ranging from 0 to 2. Linear elastic stress-deformation analysis was implemented for each μ value and; thus, 14 analyses were carried out for each screw material. ANSYS was used as an iterative technique to solve the problem. The analysis enabled the computation of the maximum von Mises equivalent stress σ_eq within each component of the assembly to compare with the yielding stress of its material.
Results
Figure 3 has presented the impact of μ on the frictional moment Mc between the bottom surface of the screw head and its contact surface with the abutment for the Ti-6Al-4V titanium alloy and type III gold screws. The difference between the wrench turning moment applied on the top surface of the head of a screw M and Mc is the moment Ms utilized to overcome friction acting on its threaded surface. For both screw materials, Mc increases with increasing μ to achieve a constant value at μ>0.5. Therefore, the moment available to overcome the thread friction decreases with μ to have a constant value at relatively higher values of μ. In the case of a gold screw, values of both Mc and Ms for all μ are invariably less than those corresponding in the case of titanium screw.
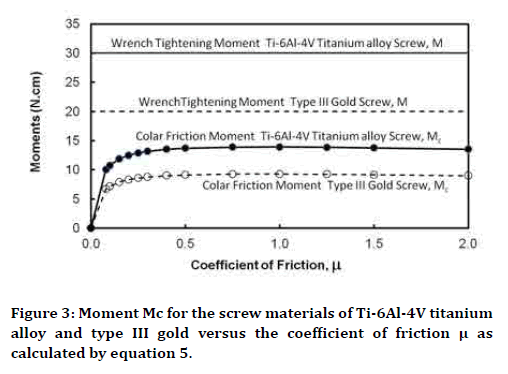
Figure 3. Moment Mc for the screw materials of Ti-6Al-4V titanium alloy and type III gold versus the coefficient of friction μ as calculated by equation 5.
Figure 4 has plotted the tightening axial pressure, Pa, against μ for the titanium and gold screws. The value of Pa starts with a relatively high value at μ=0 then decreases with increasing μ to have a constant value at higher μ values. The magnitudes of Pa in the case of the gold screw were less than those corresponding to the titanium screw for all values of μ.
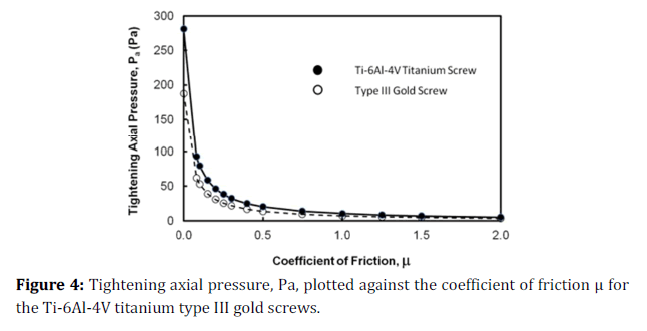
Figure 4. Tightening axial pressure, Pa, plotted against the coefficient of friction μ for the Ti-6Al-4V titanium type III gold screws.
Figure 5a has shown the variation of the maximum von Mises equivalent stress σeq with μ suffered by metallic parts of the present model in the case of titanium retaining screw. The material of the four parts represented in Figure 5a is titanium alloy. The metallic parts which have not been represented in Figure 5a have less stresses at all μ values. Generally, for the four parts presented in Figure 5a starts with a high value at μ=0 then decreases with an increase in μ to reach a constant value at a higher μ values. At relatively high μ values, the stress values generated within the parts may be arranged in a descending order as the implant upper part followed by the retaining screw, the screw collar, and finally the abutment lower part. This arrangement is different at relatively low μ values with highest stress acting on the screw collar and the lowest stress acting on the implant upper part. Similar results have been shown in Figure 5b for the case of the screw made of type III gold. In general, stresses in this case are less than those computed in the case of the titanium screw. Figures 5c and 5d have demonstrated the influence of m on the maximum value of σeq computed for the cortical and cancellous bones and the soft tissue, respectively in the cases of the titanium and gold retaining screw. With the variation of μ, the value of σeq behaves in a similar manner as described for the metallic parts of the assembly.
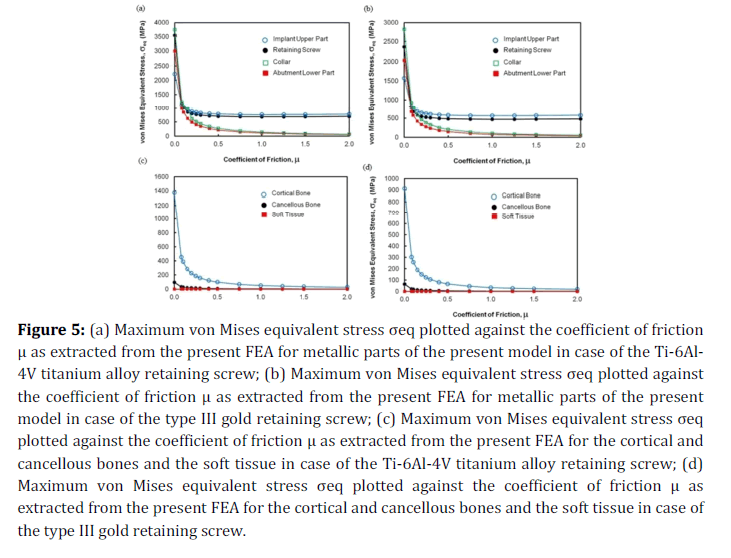
Figure 5. (a) Maximum von Mises equivalent stress σeq plotted against the coefficient of friction μ as extracted from the present FEA for metallic parts of the present model in case of the Ti-6Al-4V titanium alloy retaining screw; (b) Maximum von Mises equivalent stress σeq plotted against the coefficient of friction μ as extracted from the present FEA for metallic parts of the present model in case of the type III gold retaining screw; (c) Maximum von Mises equivalent stress σeq plotted against the coefficient of friction μ as extracted from the present FEA for the cortical and cancellous bones and the soft tissue in case of the Ti-6Al-4V titanium alloy
retaining screw; (d) Maximum von Mises equivalent stress σeq plotted against the coefficient of friction μ as extracted from the present FEA for the cortical and cancellous bones and the soft tissue in case of the type III gold retaining screw.
Discussion
The settling effect, also known as the embedment relaxation of a screw, is the result of surface machining imperfections or microscopic irregularities. These imperfections are impossible to avoid regardless of how meticulous was the machining process. Upon loading or tightening, settling occurs as these micro-irregularities flatten. Consequently, this wear of the contacting surfaces results in approximation of the surfaces being tightened [5].
Wentaschek et al. [6] evaluated the screw preload and tightening torque needed to overcome the thread friction of an uncoated titanium abutment screw complex. The screws were torqued and untorqued 10 times each. The results of the study indicated significant decrease of preload values accompanied with an increase in coefficient of friction, according to the number of repetitions.
Lang et al. [7] used finite element analysis to evaluate preload development in Nobel Bio care implant assembly systems. In the later study, the abutment screws were subjected to ascending incremental tightening torque in of 1 N cm. The effect of the coefficient of friction on delivered preload in the implant complex during and after abutment screw tightening was evaluated. The results of the study demonstrated that the use of the recommended tightening torques did not achieve the required preload that is 75% of the yield strength of the abutment screw. Analysis of stress distribution analysis demonstrated the transfer of preload force from the screw to the implant fixture during tightening. As for the effect of the coefficient of friction, the study concluded that application of the recommended torque to an abutment with a coefficient of friction value of 0.26 resulted in a less than the required preload for the abutment screws. To achieve the optimum preload value, which is of 75% of the yield strength, the value of the coefficient of friction between the implant assembly complexes must be 0.12.
To increase delivered preload to achieve the optimum values, Wu et al. [8] focused on decreasing the coefficient of friction in an in vitro laboratory and three-dimension finite element analysis. The study has used lubricant to simulate the effect of decreasing the coefficient of friction. The results of the study demonstrated that the lubricant decreased the friction coefficient, thereby, resulted in an increase in clamping force. However, the use of lubricant resulted in higher stress concentration on the screw threads. A decrease in fatigue life at high load was reported. Ductile fracture at the screw first thread was the dominant failure mode.
An accurate value of coefficient of friction for the implant assembly is not known [6]. Literature included lower coefficient of friction values because of lubrication to achieve higher tightening preload and thereby better joint stability. On the other hand, researchers also reported an increase in the coefficient of friction values with repeated tightening because of embedment relaxation. In this study, the settling effect was simulated by a range of coefficient of frictional values ranging from 0 to 2.
Jorn et al. [4] investigated the influence of lubricant on screw preload and stresses in a dental implant-abutment assembly. Friction coefficients values between 0.2 and 0.5 were used to simulate dry or lubricated conditions. Investigators demonstrated increase in screw preload with decreasing frictional coefficient. Plastic deformation at the neck of the implant was also observed with the decrease of coefficient of friction. However, no deformation was noticed in the abutment.
Conclusion
The study has concluded that the coefficient of friction is reduced to achieve tightening of the dental implant supported prosthesis assembly. However, it is not recommended to increase the delivered preload to avoid undue damaging stresses to the implant prosthesis components, as well as, the surrounding bone. The study has concluded a decrease in stresses at maximum tightening moment that is applied on the screw with increase in the coefficient of friction for all assembly parts. Adverse conditions like fractures are likely to arise because of loosening of the prosthetic retaining screw among the individuals that were not operated for retightening and movement of the prosthesis. This led to screw overload, causing deformations. Future studies need to focus on retightening and long-term use of prosthetic screw exhibiting plastic deformations.
Acknowledgment
The author is very thankful to all the associated personnel in any reference that contributed in/ for the purpose of this research. Further, this research holds no conflict of interest and is not funded through any source.
References
- Haack JE, Sakaguchi RL, Sun T, et al. Elongation and preload stress in dental implant abutment screws. Int J Oral Maxillofac Implants 1995; 10:529-36.
- Peixoto HE, Bordin D, Del Bel Cury AA, et al. The role of prosthetic abutment material on the stress distribution in a maxillary single implant-supported fixed prosthesis. Mater Sci Eng C Mater Biol Appl 2016; 65:90-96.
- Bulaqi HA, Mousavi Mashhadi M, Geramipanah F, et al. Effect of the coefficient of friction and tightening speed on the preload induced at the dental implant complex with the finite element method. J Prosthet Dent 2015; 113:405-411.
- Jorn D, Kohorst P, Besdo S, et al. Influence of lubricant on screw preload and stresses in a finite element model for a dental implant. J Prosthet Dent 2014; 112:340-348.
- Winkler S, Ring K, Ring JD et al. Implant screw mechanics and the settling effect: Overview. J Oral Implantol 2003; 29:242-45.
- Wentaschek S, Tomalla S, Schmidtmann I, et al. Coefficient of friction, and thread friction in an implant-abutment-screw complex. Int J Prosthodont 2017; 30:542-544.
- Lang LA, Kang B, Wang RF, et al. Finite element analysis to determine implant preload. J Prosthet Dent 2003; 90:539-546.
- Wu T, Fan H, Ma R, et al. Effect of lubricant on the reliability of dental implant abutment screw joint: An in vitro laboratory and three-dimension finite element analysis. Mater Sci Eng C Mater Biol Appl 2017; 75:297-304.
Author Info
Mohamed Tharwat Hamed1,2*, Hisham Abdullah Mously1, Abou Bakr Hosam Hashem3 and Ghada Hussein Naguib4,5
1Faculty of Dentistry, Department of Oral & Maxillofacial Prosthodontics, Saudi Arabia2Department of Fixed Prosthodontics, School of Dentistry, Cairo University, Egypt
3Dental Department, Research institute of Ophthalmology, Giza, Egypt
4Faculty of Dentistry, Department of Restorative Dentistry, Saudi Arabia
5Faculty of Dentistry, Department of Oral Biology, Cairo University, Egypt
Citation: Mohamed Tharwat Hamed, Hisham Abdullah Mously, Abou Bakr Hosam Hashem, Ghada Hussein Naguib, Influence of Coefficient of Friction on Stress Distribution in Implant Components and Surrounding Bone, J Res Med Dent Sci, 2019, 7(6): 48-53.
Received: 05-Sep-2019 Accepted: 08-Nov-2019