Research - (2022) Volume 10, Issue 3
The Relation between Bone Density and Implant Stability, with Osseodensification One Stage Crestal Sinus Lift: A Prospective Clinical Study
Jenna Z Alhayati and Auday M Al-Anee*
*Correspondence: Auday M Al-Anee, Department of Orthodontics, College of Dentistry, University of Baghdad, Iraq, Email:
Abstract
Background: One of the most important factors for successful osseointegration is primary stability. Many techniques have been tried in the past to improve implant primary stability in low bone density. The posterior maxilla is thought to have the lowest bone density. Furthermore, pneumatization of the maxillary sinus has a significant impact on bone height, which affects implant installation, in addition to poor density. Salah Huwais established the osseodensification technique in 2015, which employs a densifying bur to create a minimal plastic deformation. It is a unique biomechanical osteotomy preparation approach that uses a non-excavating drilling procedure to assist preserve bone. The nonexcavated compacted bone elevates and penetrates the sinus floor without perforating or breaching the membrane. Osseodensification has also improved implant stability by increasing peripheral and apical bone mineral density, bone-to-implant contact (BIC), and percentage of bone volume (BV) around it. Aim: The study is aimed to assess the relation between bone density and implant stability, with osseodensification one stage crestal sinus lift in a low-bone density atrophic posterior maxilla (residual bone height ≥ 2.0-<6.0 mm). Materials and methods: This study includes twenty crestal sinus floor elevations in seventeen individuals, with ten men and seven females meeting the qualifying criteria. A cone beam CT scan (CBCT) was performed for each participant (two weeks before surgery) to identify the exact alveolar bone height and width, as well as to record bone density at the planned implant site from the coronal view by ROI (region of interest) using On-demand software. Primary implant stability was assessed using an Osstell beacon at the time of implant placement, and secondary stability was assessed after six months of osseous healing. Results: The ages of the patients range from 29 to 70 years, with an average of 47.3 ± 11.5 years. The highest percentage of 70.6% was reported in ≥ 40 years old. Most implants were placed in D4 bone density 50%, with mean value of density 244.94 ± 70.95 HU. The highest value of primary implant stability appears in preoperative D4 bone density, and there is no statistically significant difference between primary and secondary implant stability in three groups of different bone density type. Conclusion: The current study found that a higher primary and secondary implant stability was detected in an atrophic posterior maxilla with a residual bone height of ≥ 2.0 mm and <6 mm and with a low bone density, based on the ISQ scale measured by Osstell Beacon. Furthermore, no statistically significant difference in primary and secondary implant stability exists across three different bone density types. This is due to and explains the effect of the osseodensification versah burs that increases and improves the bone density, to a proximately same range.
Keywords
Osseodensification, Crestal sinus lift, Bone density, Implant stability
Introduction
One of the most important factors for successful osseointegration is primary stability. Many techniques have been tried in the past to improve implant primary stability in low bone density. Primary stability is provided by the mechanical friction among the external implant surface and the implant osteotomy's walls [1]. Which are dependent on surgical technique, implant design (macro and micro-geometric parameters of the implant), and recipient bone characteristics (bone quality, quantity and density surrounding the implant) [2]. Mechanical primary stability led to more efficient achievement of biological secondary stability [3]. Rues et al. [4] observed that primary implant stability didn't depend on total bone thickness but tended to increase with increasing bone mineral density or overall cortical bone thickness, when they assessed bone mineral density, cortical bone thickness, and total bone thickness using micro-computed tomography (CT) of pig scapulae. For more than a decade, resonance frequency analysis (RFA) has been utilized to measure implant stability in a noninvasive, reliable, simply predictable and objective manner [5,6]. RFA has been widely utilized to measure changes in stability over time or to detect the effects of immediate or early loading [7, 8]. Meredith et al. [9] and Sennerby et al. [10], were the first to propose RFA as a very successful qualitative tool for assessing implant stability. Huang et al. [11] assessed implant behavior in various bone types and supported the reliability of RFA in stability evaluation. Friberg et al. [7] Found good reliability of the RFA in crystal torque measurements in a correlation assessment of cutting torque measurements and RFA at implant insertion. However, in a cadaver investigation, O'Sullivan et al. [5] examined insertion torque and bone characteristics and found high values for all bone types except type IV, this was consistent with the findings of Boronat Lopez et al. [12] who reported greater ISQ values for implants placed in more compact bone regions.
Misch classified bone density into five types based on the number of Hounsfield units (HU). D1 exceeds 1250 HU, D2 falls between 850 and 1250 HU, D3 falls between 350 and 850 HU, D4 falls between 150 and 350 HU, and D5 falls below 150 HU [13,14]. The most often used diagnostic method for measuring bone density is cone beam computed tomography (CBCT) [15]. Even though Hounsfield units (HU) are not directly applicable to CBCT, there has been some controversy [16]. The accuracy of CBCT for identifying trabecular bone density was compared to microcomputed tomography and multislice computed tomography (MSCT). Their findings revealed a high association between CBCT and MSCT, implying that CBCT can be utilized to determine bone mineral density at the implant site [17]. Al-Jamal and Al-Jumaily, [18] found that utilizing CBCT to determine bone density is an effective method that is linked to primary stability. Chennoju et al., [19] conclude that the CBCT was effective in calculating the original density using grey standards of CBCT scans. The posterior maxilla is thought to have the lowest bone density, with 40% D4 bone type [20]. Furthermore, pneumatization of the maxillary sinus because of posterior maxillary tooth loss has a significant impact on bone height, which affects implant installation in this location, in addition to poor density [21]. Salah Huwais established the osseodensification technique in 2015, which employs a densifying bur to create a minimal plastic deformation. It is a unique biomechanical osteotomy preparation approach that uses a non-excavating drilling procedure to assist preserve bone [22]. The nonexcavated compacted bone elevates and penetrates the sinus floor without perforating or breaching the membrane. Osseodensification (OD) has also improved implant stability by increasing peripheral and apical bone mineral density, bone-to-implant contact (BIC), and percentage of bone volume (BV) around it [23-26]. Hendi et al. propose the use of the OD method to improve bone density in low-bone density zones and show a statistically significant change in mean bone density assessed at the apical site of the implant [17].
This study is aimed to assess the relation between bone density and implant stability, with osseodensification one stage crestal sinus lift in residual bone height (RBH) of ≥ 2.0 _<6.0 mm.
Materials and Methods
The Ethics Committee of the College of Dentistry/University of Baghdad approved this prospective clinical study (protocol number: 211120). All risks connected with the proposed operation, as well as the chance of failure, were thoroughly explained to the patients, and all research participants provided signed informed permission. This study includes twenty crestal sinus floor elevations in seventeen individuals aged 29-70 years, with ten men and seven females meeting the qualifying criteria. Inclusion criteria for patient selection were: residual bone height of ≥ 2.0 <6.0 , healthy participants without any systemic disease or local condition that may affect bone healing potential, or pathological lesion at the sinus zone, and no clinical and/or radiological evidence of rhinosinusitis or other pathologies in the maxillary sinus (MS). Patients who smoked heavily (20 cigarettes per day) or who were addicted to alcohol or cocaine were excluded from the research. A cone beam CT scan (CBCT) (Kavo OP 3D Pro, Karl Kolb, Germany) was performed for each participant (2 weeks before surgery) to identify the exact alveolar bone height and width at the planned implant site. The bone density of the planned implant area was assessed using the Misch scale 2008, with the following density estimates: D1 >1250 HU, D2 850-1250 HU, D3 350-850 HU, D4 150- 350 HU, and D5 <150 HU, all recorded from the coronal view by ROI (region of interest) using On-demand software (Figure 1).
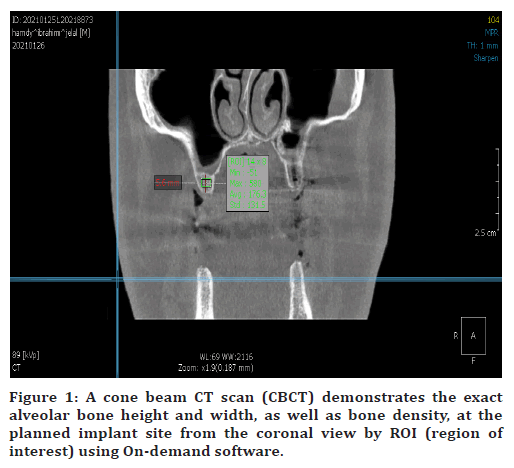
Figure 1. A cone beam CT scan (CBCT) demonstrates the exact alveolar bone height and width, as well as bone density, at the planned implant site from the coronal view by ROI (region of interest) using On-demand software.
All patients were operated on under local anesthetic by the same surgeon (Lidocaine 2% with Adrenaline 1:80,000/Septodont, France). Amoxicillin/clavulanate 1g oral tablet was administer 1 hour before surgery for antimicrobial prophylaxis. Clindamycin 600 mg was the option for allergic patients. For up to 10 days, oral rinses with Chlorhexidine digluconate mouth wash 0.2% were prescribed. A full mucoperiosteal flap (extensive or limited flap design) was reflected depending on the case demand, and drilling was started with versah® Bur 2.0 (counterclockwise drill speed 800-1500 rpm–Densifying Mode with copious irrigation), then subsequent wider versah® Bur to break and advance the past sinus floor in 1 mm increments (Maximum bur advancement past the sinus floor, must not exceed 3 mm at any stage) (Figure 2). The bone will be moved towards the apical end and will begin to slowly raise the membrane.
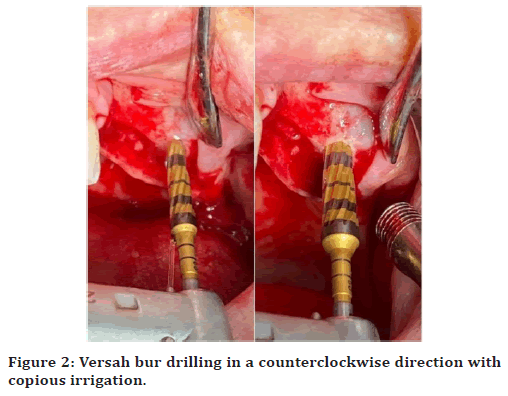
Figure 2. Versah bur drilling in a counterclockwise direction with copious irrigation.
The sinus membrane's integrity was tested by slowly injecting normal saline into the osteotomy site using a disposable plastic medical syringe filled with normal saline (the end of the needle's protective cover was cut off), If the membrane is intact, the usual saline will return to the cover (Figure 3).
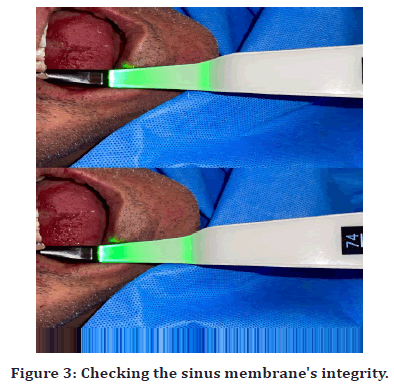
Figure 3. Checking the sinus membrane's integrity
After achieving the final desired diameter, propel a well-hydrated, alloplastic bone graft (OsteonTM II Sinus, Syringe Type/ Genoss Co., Korea) into the sinus using the final vensah® Bur in Densifying Mode (Counterclockwise) at a low speed of 150-200 rpm with no irrigation.
Endosseous Quattrocone dental implants (Medentika®, Hügelsheim, Germany) of standard sizes were used in all patients (4.3- or 5.0-mm diameter, 09 or 11 mm in length). The Osstell Beacon (Gothenburg, Sweden) employs Resonance Frequency Analysis to objectively and non-invasively measure implant stability following implant placement in the osteotomy site (primary stability). High stability is defined as greater than 70 ISQ, medium stability as 60-69 ISQ, and poor stability as 60 ISQ (Figure 4).
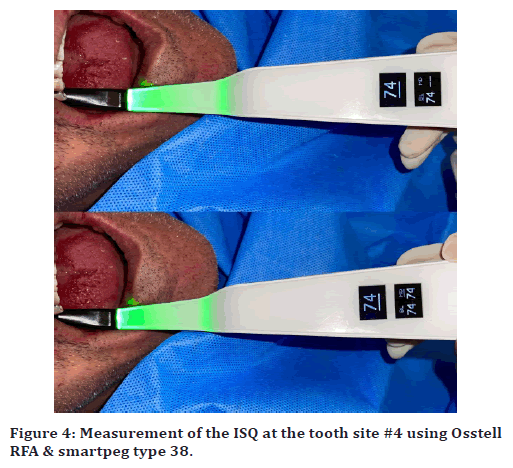
Figure 4. Measurement of the ISQ at the tooth site #4 using Osstell RFA & smartpeg type 38.
Suture removal visits were planned after ten days, clinical observation appointments after one month, and the final prosthesis and secondary implant stability record using Ostell were scheduled after six months.
Statistical analysis
Data descriptive and analysis were performed using IBM SPSS (Statistical Package for Social Sciences), software version 26. One_way Anova was used to test these data. Significant at P<0.05 not significant at P>0.05.
Results
The ages of the patients range from 29 to 70 years, with an average of 47.3 ± 11.5 years. The highest percentage of 70.6% was reported in ≥ 40 years old. In terms of gender, the proportion of males was higher than that of females, with 10 males and 7 females (58.8% vs 41.2%) respectively. According to tooth site number, the most common tooth sites were first permanent molars #3 and #14, (40% & 35%) respectively, followed by second molars (15%) and second premolar (10%). Most implants were placed in D4 bone density 50%, with mean value of density 244.94 ± 70.95 HU, followed by 25% for both D5 and D3, with the mean value of density (73.18 ± 48.38HU and 415.38 ± 17.25HU) respectively, (Table 1).
Bone density (%) | Mean (SD) | Minimum | Maximum |
---|---|---|---|
D3 (25%) | 415.38 (17.25) | 400.1 | 441.5 |
D4 (50%) | 244.94 (70.95) | 161.5 | 319.8 |
D5 (25%) | 73.18 (48.38) | 25 | 149 |
SD= Standard deviation |
Table 1: Dental implants distribution according to bone density.
In the current study, the highest value of primary implant stability appears in preoperative D4 bone density, and there is no statistically significant difference of primary and secondary implant stability in three groups of different bone density type, (Table. 2).
D3 Bone density | D4 Bone density | D5 Bone density | P-Value | |
---|---|---|---|---|
Primary stability Mean ISQ (SD) | 67.80 (12.42) | 72.70 (9.53) | 66.20 (7.07) | 0.434 [NS] |
Secondary stability Mean ISQ (SD) | 74.20 (6.22) | 73.70 (10.32) | 75.30 (5.67) | 0.943 [NS] |
SD= Standard deviation; P=probability value; NS= non-significant |
Table 2: Effect of bone density on implant stability.
Discussion
Dental implants are a functional and esthetic solution for tooth loss, especially for improving the lives of the elderly. The success of dental implants (implant survival), and implant failure is a multifactorial process that can be attributed to a variety of factors. Implant failure rates have been found to be higher when implants are placed in areas with low bone density, this is mainly due to the lower primary stability [27].
Primary stability is regarded as the first step and outcome that pave the way for biological secondary stability and osseointegration, which are dependent on bone quality, quantity and density surrounding the implant. Rues et al. [4] showed that bone density can influence primary implant stability, which is similar with the findings of De Elio Oliveros et al [2], and Farré- Pagés et al. [28]. In maxillary sinus augmentation with simultaneous implant insertion, bone density appears to be the most important factor of primary stability. Preoperative bone density measurement may aid in avoiding stabilityrelated issues in atrophic posterior maxillary implant therapy [29]. Noaman et al. demonstrates a significant positive correlation between the bone density values from CBCT and implant stability was measured using Osstell [30]. There are many ways to solve a problem of limited bone height and density of maxilla that is considered to have the lowest bone density, with 40% D4 bone type found in it [20]. The non-excavating versah burs by osseodensification technique would enhance primary stability, bone mineral density, and the percent of bone surrounding implant surface. It is theorized that by preserving bulk bone, the process of healing will be accelerated due to the bone matrix, cells, and biochemicals that are kept in place and autografted along the surface of the osteotomy site.
The principle of osseodensification is dependent on the viscoelastic nature of trabecular bone and its collagen content. When the versah bur rotates in non-cutting OD mode, the trabecular bone is preserved, compressed, and autografted, resulting in the phenomenon of spring back effect into the osteotomy center. This effect improves the implant's initial stability. The RBH range in the current study is between, ≥ 2.0 mm and <6 mm, with bone density type of D5, D4 and D3. This range is seen to be more difficult in terms of maxillary sinus floor elevation, morbidity and stability. Most implants, 50%, were classed as having D4 bone density, using on-demand software, a preoperative measurement was acquired from the CBCT coronal view. After six months of implants installation, the mean of secondary stability in the current study is significantly higher than the mean of primary stability. One such medium to high primary implant stability (based on ISQ scale) in a research sample with low bone quality and quantity is linked with the osseodensification concept. And there is no statistical significance difference of primary and secondary implant stability in three groups of different bone density types. This is due to and explains the effect of the densification versah burs that increases and improves the bone density to a proximately same range. OD will not create tissue; rather, it will optimize and preserve what already exists. To achieve a predictable plastic expansion, this procedure necessitates 2 mm of trabecular-bone core and a ≥ 1/1 trabecular: cortical bone ratio. The more cortical bone there is, the more trabecular core is required to allow for predictable expansion. The ideal minimum width of the ridge is 4mm [31]. When there are more trabecular spaces, the expansion strain is absorbed, resulting in less dimensional change and ridge expansion.
Conclusion
The current study found that a higher primary and secondary implant stability was detected in an atrophic posterior maxilla with a residual bone height of ≥ 2.0 mm and <6 mm and with a low bone density, based on the ISQ scale measured by Osstell Beacon. Furthermore, no statistically significant difference in primary and secondary implant stability exists across three different bone density types. This is due to and explains the effect of the osseodensification versah burs that increases and improves the bone density, to a proximately same range.
Conflicts of Interest
The authors have disclosed no potential conflicts of interest.
Acknowledgements
Thanks to all participants in the present study.
References
- Padhye NM, Padhye AM, Bhatavadekar NB. Osseodensification––A systematic review and qualitative analysis of published literature. J Oral Biol Craniofac Res 2020; 10:375-380.
- de Elío Oliveros J, del Canto Díaz A, del Canto Díaz M, et al. Alveolar bone density and width affect primary implant stability. J Oral Implantol 2020; 46:389-395.
- Monje A, Ravidà A, Wang HL, et al. Relationship between primary/mechanical and secondary/biological implant stability. Int J Oral Maxillofac Implant 2019; 34.
- Rues S, Schmitter M, Kappel S, et al. Effect of bone quality and quantity on the primary stability of dental implants in a simulated bicortical placement. Clin Oral Investigations 2021; 25:1265-1272.
- O'Sullivan D, Sennerby L, Meredith N. Measurements comparing the initial stability of five designs of dental implants: a human cadaver study. Clin Implant Dent Related Res 2000; 2:85-92.
- Koh RU, Rudek I, Wang HL. Immediate implant placement: Positives and negatives. Implant Dent 2010; 19:98-108.
- Friberg B, Sennerby L, Meredith N, et al. A comparison between cutting torque and resonance frequency measurements of maxillary implants: A 20-month clinical study. Int J Oral Maxillofac Surg 1999; 28:297-303.
- Javed F, Romanos GE. The role of primary stability for successful immediate loading of dental implants. A literature review. J Dent 2010; 38:612-20.
- Meredith N. Assessment of implant stability as a prognostic determinant. Int J Prosthod 1998; 11.
- Sennerby L, Meredith N. Resonance frequency analysis: measuring implant stability and osseointegration. Compendium of continuing education in dentistry (Jamesburg, NJ: 1995). 1998; 19:493-498.
- Huang HM, Lee SY, Yeh CY, et al. Resonance frequency assessment of dental implant stability with various bone qualities: A numerical approach. Clin Oral Implants Res 2002; 13:65-74.
- Boronat Lopez A. Estudio del analisis de frecuencia ´ de resonancia tras la colocacion de 133 implantes dentales. Med Oral Patol 2006; 11:272–276.
- Misch CE. Bone density: A key determinant for clinical success. Contemp. Implant Dent 1999; 8:109-18.
- Juodzbalys G, Kubilius M. Clinical and radiological classification of the jawbone anatomy in endosseous dental implant treatment. J Oral Maxillofac Res 2013; 4.
- Al-Attas MA, Koppolu P, Alanazi SA, et al. Radiographic evaluation of bone density in dentulous and edentulous patients in Riyadh, KSA. Niger J Clin Pract 2020; 23:258-265.
- Chappuis V, Araújo MG, Buser D. Clinical relevance of dimensional bone and soft tissue alterations post‐extraction in esthetic sites. Periodontol 2017; 73:73-83.
- Monje A, Monje F, González‐García R, et al. Comparison between microcomputed tomography and cone‐beam computed tomography radiologic bone to assess atrophic posterior maxilla density and microarchitecture. Clin Oral Implant Res 2014; 25:723-728.
- Al-Jamal MF, Al-Jumaily HA. Can the bone density estimated by CBCT predict the primary stability of dental implants? A new measurement protocol. J Craniofac Surg 2021; 32:e171-4.
- Chennoju SK, Ramaswamy P, Neelima V, et al. Standardization of a cone beam computed tomography machine in evaluating bone density: A novel approach. Minerva Stomatol 2020.
- Monstaporn M, Rungsiyakull C, Jia-Mahasap W, et al. Effects of bone types on bone remodeling of a dental implant: A review of the literature. Dent J 2020; 42:13-19.
- Mohan N, Wolf J, Dym H. Maxillary sinus augmentation. Dent Clin 2015; 59:375-88.
- Huwais S, Meyer EG. A novel osseous densification approach in implant osteotomy preparation to increase biomechanical primary stability, bone mineral density, and bone-to-implant contact. Int J Oral Maxillofac Implant 2017; 32.
- Yeh YT, Chu TM, Blanchard SB, et al. Effects on ridge dimensions, bone density, and implant primary stability with osseodensification approach in implant osteotomy preparation. Int J Oral Maxillofac Implant 2021; 36.
- Gaikwad AM, Joshi AA, Nadgere JB. Biomechanical and histomorphometric analysis of endosteal implants placed by using the osseodensification technique in animal models: A systematic review and meta-analysis. J Prosthet Dent 2020.
- Slete FB, Olin P, Prasad H. Histomorphometric comparison of 3 osteotomy techniques. Implant Dent 2018; 27:424-8.
- Hindi AR, Bede SY. The effect of osseodensification on implant stability and bone density: A prospective observational study. J Clin Exp Dent 2020; 12:e474.
- Herrmann I, Lekholm U, Holm S, et al. Evaluation of patient and implant characteristics as potential prognostic factors for oral implant failures. Int J Oral Maxillofac Implant 2005; 20.
- Pagés NF, Castro ML, Algarra FA, et al. Relation between bone density and primary implant stability. Med Oral Patol 2011; 16:14.
- Pommer B, Hof M, Fädler A, et al. Primary implant stability in the atrophic sinus floor of human cadaver maxillae: Impact of residual ridge height, bone density, and implant diameter. Clin Oral Implant Res 2014; 25:e109-13.
- Noaman AT, Bede SY. The effect of bone density measured by cone beam computed tomography and implant dimensions on the stability of dental implants. J Craniofac Surg 2021.
- Anitua E, Begoña L, Orive G. Clinical evaluation of split‐crest technique with ultrasonic bone surgery for narrow ridge expansion: Status of soft and hard tissues and implant success. Clin Implant Dent Related Res 2013; 15:176-87.
Indexed at, Google Scholar, Cross Ref
Indexed at, Google Scholar, Cross Ref
Indexed at, Google Scholar, Cross Ref
Indexed at, Google Scholar, Cross Ref
Indexed at, Google Scholar, Cross Ref
Indexed at, Google Scholar, Cross Ref
Indexed at, Google Scholar, Cross Ref
Indexed at, Google Scholar, Cross Ref
Indexed at, Google Scholar, Cross Ref
Indexed at, Google Scholar, Cross Ref
Indexed at, Google Scholar, Cross Ref
Indexed at, Google Scholar, Cross Ref
Indexed at, Google Scholar, Cross Ref
Indexed at, Google Scholar, Cross Ref
Indexed at, Google Scholar, Cross Ref
Indexed at, Google Scholar, Cross Ref
Indexed at, Google Scholar, Cross Ref
Indexed at, Google Scholar, Cross Ref
Indexed at, Google Scholar, Cross Ref
Indexed at, Google Scholar, Cross Ref
Indexed at, Google Scholar, Cross Ref
Indexed at, Google Scholar, Cross Ref
Indexed at, Google Scholar, Cross Ref
Author Info
Jenna Z Alhayati and Auday M Al-Anee*
Department of Orthodontics, College of Dentistry, University of Baghdad, Baghdad, IraqReceived: 04-Mar-2022, Manuscript No. JRMDS-22-57803; , Pre QC No. JRMDS-22-57803 (PQ); Editor assigned: 07-Mar-2022, Pre QC No. JRMDS-22-57803 (PQ); Reviewed: 21-Mar-2022, QC No. JRMDS-22-57803; Revised: 25-Mar-2022, Manuscript No. JRMDS-22-57803 (R); Published: 31-Mar-2022